Sustainable Chemistry
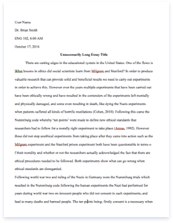
A limited time offer! Get a custom sample essay written according to your requirements urgent 3h delivery guaranteed
Order NowChemistry has an important role to play in achieving a sustainable civilization on Earth. The present economy remains utterly dependent on a massive inward flow of natural resources that includes vast amounts of nonrenewables. This is followed by a reverse flow of economically spent matter back to the ecosphere. Chemical sustainability problems are determined largely by these economy-ecosphere materials flows (see the figure, below), which current chemistry education essentially ignores. It has become an imperative* that chemists lead in developing the technological dimension of a sustainable civilization.
When chemists teach their students about the compositions, outcomes, mechanisms, controlling forces, and economic value of chemical processes, the attendant dangers to human health and to the ecosphere must be emphasized across all courses. In dedicated advanced courses, we must challenge students to conceive of sustainable processes and orient them by emphasizing through concept and example how safe processes can be developed that are also profitable. Green or sustainable chemistryâ can contribute to achieving sustainability in three key areas. First, renewable energy technologies will be the central pillar of a sustainable high-technology civilization. Chemists can contribute to the development of the economically feasible conversion of solar into chemical energy and the improvement of solar to electrical energy conversion. Second, the reagents used by the chemical industry, today mostly derived from oil, must increasingly be obtained from renewable sources to reduce our dependence on fossilized carbon. This important area is beginning to flourish, but is not the subject of this essay. Third, polluting technologies must be replaced by benign alternatives. This field is receiving considerable attention, but the dedicated research community is small and is merely scratching the surface of an immense problem that I will now sketch.
Many forces give rise to chemical pollution, but there is one overarching scientific reason why chemical technology pollutes. Chemists developing new processes strive principally to achieve reactions that only produce the desired product. This selectivity is achieved by using relatively simple reagent designs and employing almost the entire periodic table to attain diverse reactivity. In contrast, nature accomplishes a huge range of selective biochemical processes mostly with just a handful of environmentally common elements. Selectivity is achieved through a reagent design that is much more elaborate than the synthetic one. For example, electric eelscan store charge via concentration gradients of iochemically commonalkali metal ions across the membranes of electroplaque cells. In contrast, most batteries used for storing charge require biochemicallyforeign, toxic elements, such as lead and cadmium. Because of thisstrategic difference, manmade technologies often distribute throughoutthe environment persistent pollutants that are toxic because theycontain elements that are used sparingly or not at all in biochemistry.
Persistent bioaccumulative pollutants pose the greatest chemical threat to sustainability. They can be grouped into two classes. Toxic elements are the prototypical persistent pollutants; long-lived radioactive elements are especially dangerous examples. New toxicities continue to be discovered for biologically uncommon elements. The second class consists of degradation-resistant molecules. Many characterized examples originate from the chlorine industry⥠and are also potently bioaccumulative. For example, polychlorinated
dibenzo-dioxins and -furans (PCDDs and PCDFs) are deadly, persistent organic pollutants. They can form in the bleaching of wood pulp with chlorine-based oxidants, the incineration of chlorine-containing compounds and organic matter, and the recycling of metals. The United Nations Environmental Program (UNEP) International Agreement on persistent organic pollutants lists 12 âpriorityâ pollutant compounds and classes of compounds for global phaseout. All are organochlorines.
Imagine all of Earth’s chemistry as a mail sorter’s wall of letter slots in a post office, with the network of compartments extending toward infinity (see the figure, below). Each compartment represents a separate chemistry so that, for example, thousands of compartments are associated with stratospheric chemistry or with a human cell. An environmentally mobile persistent pollutant can move from compartment to compartment, sampling a large number and finding those compartments that it can perturb. Many perturbations may be inconsequential, but others can cause unforeseen catastrophes, such as the ozone hole or some of the manifestations of endocrine disruption.§ Most compartments remain unidentified and even for known compartments, the interactions of the pollutant with the compartment’s contents can usually not be foreseen, giving ample reason for scientific humility when considering the safety of persistent mobile compounds. We should heed the historical lesson that persistent pollutants are capable of environmental mayhem, and treat them with extreme caution. In cases where the use of a persistent pollutant is based on a compelling benefit, as with DDT (dichlorodiphenyltrichloroethane) in malaria-infested regions, chemists must face the challenge of finding safe alteratives.
Consider, for instance, the alarming reproductive damage that can be inflicted by minute quantities of endocrine-disrupting chemicals (EDCs), such as PCDDs, polychlorobiphenyls (PCBs), and the pesticides endosulfan and atrazine.⥠EDCs disrupt the body’s natural control over the reproductive system by mimicking or blocking the regulatory functions of the steroid hormones or altering the amounts of hormones in the body. Uncertainty still clouds our understanding of their full impact, but mass sterilization is one limiting conceivable outcome of ignoring the demonstrated dangers of EDCs. Our present knowledge strongly suggests that anthropogenic EDCs should be identified and eliminated altogether.
Stringent regulations based on the precautionary principle and the principle of âreversed onusâ⥠should be developed to guard against the release of new environmentally mobile persistent compounds; a precise definition of persistence also needs to be developed. This would provide a regulatory foundation for weeding persistent bioaccumulative compounds out of all technology, and highlight where research is needed to find safe alternatives. Groundbreaking legislative proposals toward this goal are about to be considered in the Swedish Parliament.
In their current formal training, all chemistry students will learn that the chlorination of phenol proceeds by a mechanism known as electrophilic aromatic substitution. But very few will learn of EDCs and their dangers or come to know that prime examples of EDCs, namely PCDDs, are produced in trace quantities whenever phenol is chlorinated. This hazardous omission illustrates one important type of content that is simply missing from the conventional curriculum.
Green chemistry can dramatically reduce environmental burdens of both classes of persistent pollutants by moving the elemental balance of technology closer to that of biochemistry. Significant reductions in the dispersal of many persistent pollutants have already been achieved. By the late 17th century, the use of lead oxide as a correcting agent for acidic wine was banned on pain of death in Ulm in the duchy of Wurtemburg.| More recently, large reductions in lead pollution have been achieved in what are recognizable examples of green chemistry, for instance, by replacement of lead additives in paint with safe alternatives, by the development of cleaner batteries, and by the as yet unfinished and sometimes flawed progression away from tetraethyl lead toward safer combustion promoters in fuels. PCDDs and PCDFs have been greatly reduced in the pulp and paper industry by the replacement of chlorine with chlorine dioxide as the principal bleaching agent.
Nevertheless, much more can and must be done. For example, chlorine-based oxidations such as pulp bleaching, water disinfection, household and institutional cleaning, and clothing care continue to produce huge volumes of organochlorine-containing effluent. Despite industry efforts to reduce pollutant concentrations, some of the inescapable trace contaminants are persistent, bioaccumulative carcinogens and/or EDCs. Chlorine-based oxidation technologies could be replaced with alternatives based on catalytic activation of nature’s principal oxidizing agents, oxygen or hydrogen peroxide. My research group has patented TAML activators, which are potent but selective peroxide-activating catalysts comprised of biochemically common elements for these and other fields of use. Environmental considerations also underpin the worldwide investigation and development of supercritical and near-critical carbon dioxide as a clean solvent. The present search for safer solvents in the green chemistry community is distinguished by a remarkable burst of creativity that perhaps reaches its zenith in ionic liquids. These solvents have unique properties such as the absence of any vapor pressure under standard conditions.
Pollutant production can also be reduced by improving process selectivity, reducing energy intensity, and minimizing the flow of matter to and from the ecosphere via atom economic processes, that is, processes optimized to reduce per unit of product the quantities of chemicals employed in the reactions as solvents and reagents or produced as by-products.
To achieve such sustainable chemistry requires a sea change in the chemical community. The principles of green or sustainable chemistry must become an integral part of chemical education and practice. However, there are several obstacles to overcome. First, chemists need to comprehensively incorporate environmental considerations into their decisions concerning the reactions and technologies to be developed in the laboratory. These questions need to become as important as those associated with the selectivity of the technology and how it works. Principles upon which to base these decisions have already been developed.â Second, it is critical that chemistry that is not really green does not get sold as such, and that the public is not misled with false or insufficient safety information. For example, certain chlorine industry companies have sought to protect their profits by distorting scientific data to make dioxins appear to be less harmful to humans than they actually are.⥠The general trust that chemical risk is treated in a fair and reasonable manner must be strengthened. Third, since many chemical sustainability goals such as those associated with solar energy conversion call for ambitious, highly creative research approaches, short-term and myopic thinking must be avoided. Government, universities, and industry must learn to value and support research programs that do not rapidly produce
publications, but instead present reasonable promise of promoting sustainability. Fourth, chemistry exerts a near boundless influence on human action and is thus inextricably intertwined with ethics. An understanding of sustainability ethics* is therefore an essential component of a healthy chemical education.
The all-encompassing challenge lying before green chemists is to understand the ethical forces, chemical-ecosphere relations, educational needs, and research imperatives that sustainability brings center stage and to reconcile this understanding as much as possible with economic maxims. If chemists increasingly direct their strengths to contributing to a sustainable civilization, chemistry will become more interesting and compelling to people, and may lose its âtoxicâ image. It will become more worthy of public support and spawn exciting economic enterprises that nurture sustainability.
Green & Sustainable Chemistry
1. Concept of Green & Sustainable Chemistry
Those who work in the area of chemistry and chemical technology shall recognize their responsibility to the society as members thereof, place the fundamental priority of their activity on Environment and Human Health and Safety in pursuit of the ultimate goal, and contribute to realize Sustainable Society, through Innovation of Chemical Technology.
2. Definition of Green & Sustainable Chemistry
Green & Sustainable Chemistry is defined as “Chemical Technologies to realize the Human and Environmental Health, minimization of energy and resource consumption and others, through innovations and improvements in product and process design, selection of feedstocks, formulations and applications and resource recycling”. The whole life cycle of chemical product should be taken into account for every step of production.
3. Key-factors promoting Green & Sustainable Chemistry 1) Technologies for pollution prevention based on holstic environment impact assessment.
2) Innovative technologies with wide and practical applicability. 3) Global and international contribution. 4) Conditions surrounding Japanese society, such as environment, resources and demographic change.
5) Planning and actions with long term strategies and dynamic responses to changing society and global situations.
4. Guidelines for Practicing Green & Sustainable Chemistry 1) Pursuance of highly effective and economical product and process design and technologies in consideration of holistic life cycle assessment and the environment and human health and safety.
2) Development of products and technologies which contribute to minimize by-products, emmision chemicals, residues and wastes, recycling of materials to resources and cleaning remediation of environment.
3) Development of combined multiple technological and operational systems for reduction of energy and resources and cyclic ultilization of materials and chemicals.
4) Reduction in dependency on non-renewable feedstocks by promoting utilization of renewable feedstocks and renewing technologies. 5) Promotion of collaboration and joint works among industry. academia and national institutes, other industries and various disciplines, both in domestically and internationally.
6) Promotion of information exchange, dissemination and communication to enhance reliability of chemistry among the society.
7) Promotion of education and enlightening in regards to Green & Sustainable Chemistry to achieve its ultimate goal, i.e. realization of sustainable society.
What is sustainable chemistry or green chemistry? It is normally recognized as a chemical philosophy of designing environmentally friendly processes and minimizing waste or pollution. Biotechnology also contributes in creating green chemistry. One project which the Peking University research team has been working on for the International Genetically Engineered Machine competition (iGEM 2010 Peking Team), is developing a bacteria-based heavy metal detecting, accumulating and recycling bio-kit, which is an example of realizing green chemistry goals through utilizing biological methods.
However, green chemistry is much more than simply reducing waste or improving the efficiency of reactions. It also includes fundamental scientific discoveries which in turn enhance the âgreen-nessâ of other fields. Through effective interaction with related areas, the sustainable nature of green chemistry may now be found throughout the developed world, becoming a routine aspect of daily life.
Our project for the Dow Sustainability Innovation Student Challenge focuses on sustainability within the human body itself, which is, sustainable healthcare. Sustainable healthcare, or ecologically sustainable medicine (ESM), is defined by the long-term maintenance of health which depends on the regular maintenance of environmental health and the rational use of natural resources. As sustainable chemistry is based on the concept of âminimizing waste by not producing it at the source,â the major purpose of sustainable healthcare is to prevent disease beforehand instead of curing it afterwards. This coincides with the time-honored Chinese saying: âThe greatest doctor cures disease before it ever develops.â
The two primary principles in sustainable healthcare are as follows:
1. Medical treatment aims at maximizing human health. This requires a comprehensive understanding of new medical technologies. It makes use of its positive effects while minimizing its negative effects. For example, the widespread drug-resistant bacterial infection caused by the overuse of antibiotics is a side-effect of inadequately
considering the negative impact of antibiotics.
2. Medical treatment is economically sustainable, i.e., the cost is minimized. Medical science develops relatively inexpensive, efficient drugs and treatment to meet the basic needs of all patients. The focus of medicine also shifts from curing to the prevention of disease. For example, the conventional treatment for diabetic patients is long-term insulin medication, rather than addressing the root cause of diabetes. That burdens patients both physically and financially through solely focusing on the disease itself while ignoring the patientâs fundamental needs.
In our project, we demonstrate two examples for each principle of sustainable healthcare, both realized by a result of chemical research.
Reactive oxygen species (ROS) are regarded as the root cause of many chronic diseases. Among them, organic hydroperoxides (OHPs) are the major agent responsible for most types of damage in the human body, which may be regarded as a conglomerate of organic molecules. We developed a protein-based probe, OHSer, to selectively detect OHPs in vivo. Its value in sustainable healthcare has been demonstrated by two specific applications.
Antibiotic resistance
Antibiotic resistance has been recognized as one of the worldâs most pressing public health problems. According to the U.S. Centers for Disease Control and Prevention, antibiotic resistant infections in the United States are responsible for $20 billion excess healthcare costs, $35 billion societal costs and eight million additional hospital days. This problem is more severe in China, with mortality in the hundreds of thousands and annual increases in the numbers of handicapped patients due to such infections. The key for treating antibiotic resistant infections is to use appropriate types and dosages of antibiotics according to the varying characteristics of the different infections, instead of overdosing as a standard protocol.
We developed a system for optimizing the antibiotic selection process for clinical practitioners. Utilizing OHSer, we observed that bacteria antibiotic resistance was directly linked with their OHP production when drugged. Therefore, when dealing with a specific kind of infection, we initially determined the minimal effective concentration of various antibiotics. We subsequently exposed bacteria to the antibiotic sets and screened the amount of bacterial OHP production utilizing OHSer. The antibiotic which induces the least OHP production is the optimal one, as it is least likely to trigger resistance. Accordingly, clinical practitioners will be able to prescribe the appropriate type and dosage of antibiotic according to the different kinds of infection.
Type 2 diabetes
Diabetes is the worldâs ninth leading cause of death, with such potentially lethal complications as stroke and coronary disease. According to WHO, 346 million patients worldwide are afflicted with diabetes, including 25.8 million in the United States. More than 80 percent of diabetes deaths occur in low- and middle-income countries. China has the dubious distinction of having the greatest number of diabetes patients, 100 million.
Type 2 diabetes accounts for more than 90 percent of all diagnosed cases of diabetes. Its characteristics include impaired glucose metabolism and pancreatic dysfunction. Through the use of OHSer, we discovered that a high concentration of glucose significantly increased the OHP level in cell nuclei, causing cellular abnormality and apoptosis. Therefore, the âsilver bulletâ for targeting the root cause rather than the symptoms of diabetes may be an effective nucleus-targeting antioxidant treatment. By protecting nuclear DNA from oxidative damage from the outset, subsequent cell death and organ dysfunction may be avoided, and the progression of diabetes may be eventually prevented or reversed. Effective antioxidants would be efficiently selected by screening with OHSer. Such treatment would likely cost much less than life-long insulin medication and would certainly be far more convenient from the perspective of patients.
The principles of green chemistry are directly tied to sustainable healthcare as well as to cognate fields linked to human life.
Think ahead, beware in time and keep in mind: prevention is more sustainable than solution. Thatâs the core of a sustainable life.
Zhao Boxuan is an undergraduate student at the College of Chemistry and Molecular Engineering at Peking University, China. His research interests include protein engineering and the physiological roles of small molecules. He is the president of the Life Sciences Industry Association of Peking University, an active student association dedicated to bridging the gap between university students and industry professionals, as well as advocating green ideas and technology. Boxuan was honored with the Dow Sustainability Innovation Student Challenge Awards at Peking University in 2011.
âHydrogen fuel is one of the most hotly-pursued challenges in chemistry’
HUGE POTENTIAL:Creative teaching is the key to encouraging students to opt for chemistry.
Bangalore: Chemistry, India’s best performing subject, promises solutions to some of today’s most pressing problems, including sustainable energy and climate change, said C.N.R. Rao, Chairman of the Scientific Advisory Council to the Prime Minister.
Speaking to presspersons on the eve of the International Year of Chemistry here on Friday, Prof. Rao said that hydrogen-fuelled cars and artificial photosynthesis to combat carbon emissions could be some of the most important chemistry-led innovations in the coming decades.
One of the most hotly-pursued challenges in chemistry is the creation of sustainable hydrogen power by splitting water molecules. âWhat we need today is an efficient method to store this energy, in automobiles for instance,â he said.
Jawaharlal Nehru Centre for Advanced Scientific Research (of which Prof. Rao is honorary president) is working towards using graphene to store hydrogen. âHere hydrogen can be released using ultraviolet light,â he said.
He added that Ratan Tata, Chairman of the Tata Group, has invested $15 million in a start-up company in the United States which is working towards splitting hydrogen from water and storing hydrogen fuel.
Despite the exciting research opportunities that chemistry presents, the subject is âunder-recognisedâ in India and often taught in a ârestrictive mannerâ, Prof. Rao said and added that creative teaching is the key to encouraging students to opt for the subject.
Chemistry’s year
International Year of Chemistry (2011) coincides with the centenary of the first Nobel Prize awarded to a woman chemist, Marie Curie, for her discovery
of radioactivity.
The Chemical Research Society of India will organise several programmes to celebrate the International Year of Chemistry under the theme âChemistry, our life, our futureâ, according to V. Krishnan, Chemical Research Society of India president.
Toward Sustainable Chemistry
Terry Collins*
Chemistry has an important role to play in achieving a sustainable civilization on Earth. The present economy remains utterly dependent on a massive inward flow of natural resources that includes vast amounts of nonrenewables. This is followed by a reverse flow of economically spent matter back to the ecosphere. Chemical sustainability problems are determined largely by these economy-ecosphere material flows (see the figure, below), which current chemistry education essentially ignores. It has become an imperative* that chemists lead in developing the technological dimension of a sustainable civilization.
When chemists teach their students about the compositions, outcomes, mechanisms, controlling forces, and economic value of chemical processes, the attendant dangers to human health and to the ecosphere must be emphasized across all courses. In dedicated advanced courses, we must challenge students to conceive of sustainable processes and orient them by emphasizing through concept and example how safe processes can be developed that are also profitable.
Green or sustainable chemistry=E2=80=A0 can contribute to achieving – Show quoted text – sustainability in three key areas. First, renewable energy technologies will be the central pillar of a sustainable high-technology civilization. Chemists can contribute to the development of the economically feasible conversion of solar into chemical energy and the improvement of solar to electrical energy conversion. Second, the reagents used by the chemical industry, today mostly derived from oil, must increasingly be obtained from renewable sources to reduce our dependence on fossilized carbon. This important area is beginning to flourish, but is not the subject of this essay. Third, polluting technologies must be replaced by benign alternatives. This field is receiving considerable attention, but the dedicated research community is small and is merely scratching the surface of an immense problem that I will now sketch.
Many forces give rise to chemical pollution, but there is one overarching scientific reason why chemical technology pollutes. Chemists developing new processes strive principally to achieve reactions that only produce the desired product. This selectivity is achieved by using relatively simple reagent designs and employing almost the entire periodic table to attain diverse reactivity. In contrast, nature accomplishes a huge range of selective biochemical processes mostly with just a handful of environmentally common elements. Selectivity is achieved through a reagent design that is much more elaborate than the synthetic one. For example, electric eels can store charge via concentration gradients of biochemically common alkali metal ions across the membranes of electroplaque cells. In contrast, most batteries used for storing charge require biochemically foreign, toxic elements, such as lead and cadmium. Because of this strategic difference, manmade technologies often distribute throughout the environment persistent pollutants that are toxic because they contain elements that are used sparingly or not at all in biochemistry.
Persistent bioaccumulative pollutants pose the greatest chemical threat to sustainability. They can be grouped into two classes. Toxic elements are the prototypical persistent pollutants; long-lived radioactive elements are especially dangerous examples. New toxicities continue to be discovered for biologically uncommon elements. The second class consists of degradation-resistant molecules. Many characterized examples originate from the chlorine industry=E2=80=A1 and ar= e also potently bioaccumulative. For example, polychlorinated dibenzo-dioxins and -furans (PCDDs and PCDFs) are deadly, persistent organic pollutants. They can form in the bleaching of wood pulp with chlorine-based oxidants, the incineration of chlorine-containing compounds and organic matter, and the recycling of metals. The United Nations Environmental Program (UNEP) International Agreement on persistent organic pollutants lists 12 =E2=80=9Cpriority=E2=80=9D pollutant= compounds and classes of compounds for global phaseout. All are organochlorines.
Imagine all of Earth’s chemistry as a mail sorter’s wall of letter slots in a post office, with the network of compartments extending toward infinity (see the figure, below). Each compartment represents a separate chemistry so that, for example, thousands of compartments are associated with stratospheric chemistry or with a human cell. An environmentally mobile persistent pollutant can move from compartment to compartment, sampling a large number and finding those compartments that it can perturb. Many perturbations may be inconsequential, but others can cause unforeseen catastrophes, such as the ozone hole or some of the manifestations of endocrine disruption.=C2=A7 Most compartments remain unidentified and even for known compartments, the interactions of the pollutant with the compartment’s contents can usually not be foreseen, giving ample reason for scientific humility when considering the safety of persistent mobile compounds. We should heed the historical lesson that persistent pollutants are capable of environmental mayhem, and treat them with extreme caution. In cases where the use of a persistent pollutant is based on a compelling benefit, as with DDT (dichlorodiphenyltrichloroethane) in malaria-infested regions, chemists must face the challenge of finding safe alteratives.
Consider, for instance, the alarming reproductive damage that can be inflicted by minute quantities of endocrine-disrupting chemicals (EDCs), such as PCDDs, polychlorobiphenyls (PCBs), and the pesticides endosulfan and atrazine.=E2=80=A1 EDCs disrupt the body’s natural control o= ver the reproductive system by mimicking or blocking the regulatory functions of the steroid hormones or altering the amounts of hormones in the body. Uncertainty still clouds our understanding of their full impact, but mass sterilization is one limiting conceivable outcome of ignoring the demonstrated dangers of EDCs. Our present knowledge strongly suggests that anthropogenic EDCs should be identified and eliminated altogether.
Stringent regulations based on the precautionary principle and the principle of =E2=80=9Creversed onus=E2=80=9D=E2=80=A1 should be developed t= – Show quoted text – o guard against the release of new environmentally mobile persistent compounds; a precise definition of persistence also needs to be developed. This would provide a regulatory foundation for weeding persistent bioaccumulative compounds out of all technology, and highlight where research is needed to find safe alternatives. Groundbreaking legislative proposals toward this goal are about to be considered in the Swedish Parliament.
In their current formal training, all chemistry students will learn that the chlorination of phenol proceeds by a mechanism known as electrophilic aromatic substitution. But very few will learn of EDCs and their dangers or come to know that prime examples of EDCs, namely PCDDs, are produced in trace quantities whenever phenol is chlorinated. This hazardous omission illustrates one important type of content that is simply missing from the conventional curriculum.
Green chemistry can dramatically reduce environmental burdens of both classes of persistent pollutants by moving the elemental balance of technology closer to that of biochemistry. Significant reductions in the dispersal of many persistent pollutants have already been achieved. By the late 17th century, the use of lead oxide as a correcting agent for acidic wine was banned on pain of death in Ulm in the duchy of Wurtemburg.| More recently, large reductions in lead pollution have been achieved in what are recognizable examples of green chemistry, for instance, by replacement of lead additives in paint with safe alternatives, by the development of cleaner batteries, and by the as yet unfinished and sometimes flawed progression away from tetraethyl lead toward safer combustion promoters in fuels. PCDDs and PCDFs have been greatly reduced in the pulp and paper industry by the replacement of chlorine with chlorine dioxide as the principal bleaching agent.
Nevertheless, much more can and must be done. For example, chlorine-based oxidations such as pulp bleaching, water disinfection, household and institutional cleaning, and clothing care continue to produce huge volumes of organochlorine-containing effluent. Despite industry efforts to reduce pollutant concentrations, some of the inescapable trace contaminants are persistent, bioaccumulative carcinogens and/or EDCs. Chlorine-based oxidation technologies could be replaced with alternatives based on catalytic activation of nature’s principal oxidizing agents, oxygen or hydrogen peroxide. My research group has patented TAML activators, which are potent but selective peroxide-activating catalysts comprised of biochemically common elements for these and other fields of use. Environmental considerations also underpin the worldwide investigation and development of supercritical and near-critical carbon dioxide as a clean solvent. The present search for safer solvents in the green chemistry community is distinguished by a remarkable burst of creativity that perhaps reaches its zenith in ionic liquids. These solvents have unique properties such as the absence of any vapor pressure under standard conditions.
Pollutant production can also be reduced by improving process selectivity, reducing energy intensity, and minimizing the flow of matter to and from the ecosphere via atom economic processes, that is, processes optimized to reduce per unit of product the quantities of chemicals employed in the reactions as solvents and reagents or produced as by-products.
To achieve such sustainable chemistry requires a sea change in the chemical community. The principles of green or sustainable chemistry must become an integral part of chemical education and practice. However, there are several obstacles to overcome. First, chemists need to comprehensively incorporate environmental considerations into their decisions concerning the reactions and technologies to be developed in the laboratory. These questions need to become as important as those associated with the selectivity of the technology and how it works. Principles upon which to base these decisions have already been developed.=E2=80=A0 Second, it is critical that chemistry that is not reall= y green does not get sold as such, and that the public is not misled with false or insufficient safety information. For example, certain chlorine industry companies have sought to protect their profits by distorting scientific data to make dioxins appear to be less harmful to humans than they actually are.=E2=80=A1 The general trust that chemical risk is treated in a fair and reasonable manner must be strengthened. Third, since many chemical sustainability goals such as those associated with solar energy conversion call for ambitious, highly creative research approaches, short-term and myopic thinking must be avoided. Government, universities, and industry must learn to value and support research programs that do not rapidly produce
publications, but instead present reasonable promise of promoting sustainability. Fourth, chemistry exerts a near boundless influence on human action and is thus inextricably intertwined with ethics. An understanding of sustainability ethics* is therefore an essential component of a healthy chemical education.
The all-encompassing challenge lying before green chemists is to understand the ethical forces, chemical-ecosphere relations, educational needs, and research imperatives that sustainability brings center stage and to reconcile this understanding as much as possible with economic maxims. If chemists increasingly direct their strengths to contributing to a sustainable civilization, chemistry will become more interesting and compelling to people, and may lose its =E2=80=9Ctoxic= =E2=80=9D image. It will become more worthy of public support and spawn exciting economic enterprises that nurture sustainability.
Green & Sustainable Chemistry
1. Concept of Green & Sustainable Chemistry
Those who work in the area of chemistry and chemical technology shall recognize their responsibility to the society as members thereof, place the fundamental priority of their activity on Environment and Human Health and Safety in pursuit of the ultimate goal, and contribute to realize Sustainable Society, through Innovation of Chemical Technology.
2. Definition of Green & Sustainable Chemistry
Green & Sustainable Chemistry is defined as “Chemical Technologies to realize the Human and Environmental Health, minimization of energy and resource consumption and others, through innovations and improvements in product and process design, selection of feedstocks, formulations and applications and resource recycling”. The whole life cycle of chemical product should be taken into account for every step of production.
3. Key-factors promoting Green & Sustainable Chemistry 1) Technologies for pollution prevention based on holstic environment impact assessment.
2) Innovative technologies with wide and practical applicability. 3) Global and international contribution. 4) Conditions surrounding Japanese society, such as environment, resources and demographic change.
5) Planning and actions with long term strategies and dynamic responses to changing society and global situations.
4. Guidelines for Practicing Green & Sustainable Chemistry 1) Pursuance of highly effective and economical product and process design and technologies in consideration of holistic life cycle assessment and the environment and human health and safety.
2) Development of products and technologies which contribute to minimize by-products, emmision chemicals, residues and wastes, recycling of materials to resources and cleaning remediation of environment.
3) Development of combined multiple technological and operational systems for reduction of energy and resources and cyclic ultilization of materials and chemicals.
4) Reduction in dependency on non-renewable feedstocks by promoting utilization of renewable feedstocks and renewing technologies. 5) Promotion of collaboration and joint works among industry. academia and national institutes, other industries and various disciplines,
both in domestically and internationally.
6) Promotion of information exchange, dissemination and communication to enhance reliability of chemistry among the society.
7) Promotion of education and enlightening in regards to Green & Sustainable Chemistry to achieve its ultimate goal, i.e. realization of sustainable society.
What is sustainable chemistry or green chemistry? It is normally recognized as a chemical philosophy of designing environmentally friendly processes and minimizing waste or pollution. Biotechnology also contributes in creating green chemistry. One project which the Peking University research team has been working on for the
International Genetically Engineered Machine competition (iGEM 2010 Peking Team), is developing a bacteria-based heavy metal detecting, accumulating and recycling bio-kit, which is an example of realizing green chemistry goals through utilizing biological methods.
However, green chemistry is much more than simply reducing waste or improving the efficiency of reactions. It also includes fundamental scientific discoveries which in turn enhance the =E2=80=9Cgreen-ness=E2=80= =9D of other
fields. Through effective interaction with related areas, the sustainable nature of green chemistry may now be found throughout the developed world, becoming a routine aspect of daily life.
Our project for the Dow Sustainability Innovation Student Challenge focuses on sustainability within the human body itself, which is, sustainable healthcare. Sustainable healthcare, or ecologically sustainable medicine (ESM), is defined by the long-term maintenance of health which depends on the regular maintenance of environmental health and the rational use of natural resources. As sustainable chemistry is based on the concept of =E2=80=9Cminimizing waste by not producing it at the source,=E2=80=9D the major purpose of sustainable healthcare is to prevent disease beforehand instead of curing it afterwards. This coincides with the time-honored Chinese saying: =E2=80=9CT= he greatest doctor cures disease before it ever develops.=E2=80=9D
The two primary principles in sustainable healthcare are as follows:
1. Medical treatment aims at maximizing human health. This requires a comprehensive understanding of new medical technologies. It makes use of its positive effects while minimizing its negative effects. For example, the widespread drug-resistant bacterial infection caused by the overuse of antibiotics is a side-effect of inadequately
considering the negative impact of antibiotics.
2. Medical treatment is economically sustainable, i.e., the cost is minimized. Medical science develops relatively inexpensive, efficient drugs and treatment to meet the basic needs of all patients. The focus of medicine also shifts from curing to the prevention of disease. For example, the conventional treatment for diabetic patients is long-term insulin medication, rather than addressing the root cause of diabetes. That burdens patients both physically and financially through solely focusing on the disease itself while ignoring the patient=E2=80=99s fundamental needs.
In our project, we demonstrate two examples for each principle of sustainable healthcare, both realized by a result of chemical research.
Reactive oxygen species (ROS) are regarded as the root cause of many chronic diseases. Among them, organic hydroperoxides (OHPs) are the major agent responsible for most types of damage in the human body, which may be regarded as a conglomerate of organic molecules. We developed a protein-based probe, OHSer, to selectively detect OHPs in vivo. Its value in sustainable healthcare has been demonstrated by two specific applications.
Antibiotic resistance
Antibiotic resistance has been recognized as one of the world=E2=80=99s mos= t pressing public health problems. According to the U.S. Centers for Disease Control and Prevention, antibiotic resistant infections in the United States are responsible for $20 billion excess healthcare costs, $35 billion societal costs and eight million additional hospital days. This problem is more severe in China, with mortality in the hundreds of thousands and annual increases in the numbers of handicapped patients due to such infections. The key for treating antibiotic resistant infections is to use appropriate types and dosages of antibiotics according to the varying characteristics of the different infections, instead of overdosing as a standard protocol.
We developed a system for optimizing the antibiotic selection process for clinical practitioners. Utilizing OHSer, we observed that bacteria antibiotic resistance was directly linked with their OHP production when drugged. Therefore, when dealing with a specific kind of infection, we initially determined the minimal effective concentration of various antibiotics. We subsequently exposed bacteria to the antibiotic sets and screened the amount of bacterial OHP production utilizing OHSer. The antibiotic which induces the least OHP production is the optimal one, as it is least likely to trigger resistance. Accordingly, clinical practitioners will be able to prescribe the appropriate type and dosage of antibiotic according to the different kinds of infection.
Type 2 diabetes
Diabetes is the world=E2=80=99s ninth leading cause of death, with such potentially lethal complications as stroke and coronary disease. According to WHO, 346 million patients worldwide are afflicted with diabetes, including 25.8 million in the United States. More than 80 percent of diabetes deaths occur in low- and middle-income countries. China has the dubious distinction of having the greatest number of diabetes patients, 100 million.
Type 2 diabetes accounts for more than 90 percent of all diagnosed cases of diabetes. Its characteristics include impaired glucose metabolism and pancreatic dysfunction. Through the use of OHSer, we discovered that a high concentration of glucose significantly increased the OHP level in cell nuclei, causing cellular abnormality and apoptosis. Therefore, the =E2=80=9Csilver bullet=E2=80=9D for targeting= the root
cause rather than the symptoms of diabetes may be an effective nucleus-targeting antioxidant treatment. By protecting nuclear DNA from oxidative damage from the outset, subsequent cell death and organ dysfunction may be avoided, and the progression of diabetes may be eventually prevented or reversed. Effective antioxidants would be efficiently selected by screening with OHSer. Such treatment would likely cost much less than life-long insulin medication and would certainly be far more convenient from the perspective of patients.
The principles of green chemistry are directly tied to sustainable healthcare as well as to cognate fields linked to human life.
Think ahead, beware in time and keep in mind: prevention is more sustainable than solution. That=E2=80=99s the core of a sustainable life.
Zhao Boxuan is an undergraduate student at the College of Chemistry and Molecular Engineering at Peking University, China. His research interests include protein engineering and the physiological roles of small molecules. He is the president of the Life Sciences Industry Association of Peking University, an active student association dedicated to bridging the gap between university students and industry professionals, as well as advocating green ideas and technology. Boxuan was honored with the Dow Sustainability Innovation Student Challenge Awards at Peking University in 2011.
HUGE POTENTIAL:Creative teaching is the key to encouraging students to opt for chemistry.
Bangalore: Chemistry, India’s best performing subject, promises solutions to some of today’s most pressing problems, including sustainable energy and climate change, said C.N.R. Rao, Chairman of the Scientific Advisory Council to the Prime Minister.
Speaking to presspersons on the eve of the International Year of Chemistry here on Friday, Prof. Rao said that hydrogen-fuelled cars and artificial photosynthesis to combat carbon emissions could be some of the most important chemistry-led innovations in the coming decades.
One of the most hotly-pursued challenges in chemistry is the creation of sustainable hydrogen power by splitting water molecules. =E2=80=9CWhat w= e need today is an efficient method to store this energy, in automobiles for instance,=E2=80=9D he said.
Jawaharlal Nehru Centre for Advanced Scientific Research (of which Prof. Rao is honorary president) is working towards using graphene to store hydrogen. =E2=80=9CHere hydrogen can be released using ultraviolet light,=E2=80=9D he said.
He added that Ratan Tata, Chairman of the Tata Group, has invested $15 million in a start-up company in the United States which is working towards splitting hydrogen from water and storing hydrogen fuel.
Despite the exciting research opportunities that chemistry presents, the subject is =E2=80=9Cunder-recognised=E2=80=9D in India and often taught= in a =E2=80=9Crestrictive manner=E2=80=9D, Prof. Rao said and added that creativ= e teaching is the key to encouraging students to opt for the subject.