The Criticality Accident in SAROV
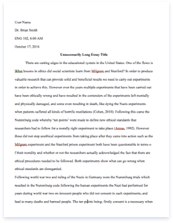
A limited time offer! Get a custom sample essay written according to your requirements urgent 3h delivery guaranteed
Order Now- Introduction
- Problem Statement
In such normal conditions, nuclear reactors are most of the time kept under a critical mass. Propagating nuclear reaction only emerges when the minimum threshold content capacity of nuclear material is reached, which usually occurs with the significant presence of a fissile material known as uranium-235 (U-235). According to Keyes (2004), U-235 atom possesses a correct geometrical configuration that can initiate chain reaction when bombarded with thermal neutron, which consequently splits the U-235 atom and creates fragments of new elements with (1) two radioactive and/or non-radioactive, (2) increased energy, and (3) three new free neutrons. Under this principle, the free neutrons further splits and cause further cascading chain reaction and uncontrolled fission, which descriptively defines the occurrence of criticality accident (Musolino 2001). One of the most highlighted accidents involving the propagation of neutron reaction, or simply criticality incident, is the happening at Sarov, Nuclear Centre, in the Russian Federation.
On June 171997, a physicist, violating the safety codes of the centre, has encountered a deadly incident during the assembly of high enriched uranium (Khodalev, Trasova and Zhinik, et al. 1998). The events of the accident have triggered tremendous physiological and pathological variations. The direct initial exposure to high neutron dose of 10-11 Gy has reached the approximation of 200-300 Gy in 10 hours based on documented medical records, which eventually led to the death of the senior technician in less than three days despite of immediate medical action.
The organization of International Atomic Energy Association (IAEA) has participated in the prompt medical action of possibly saving the life of the victim. Under the 1986 Convention on Assistance in the Case of a Nuclear Accident or Radiological Emergency to provide the Clinical Department of the Institute of Biophysics of the Ministry of Health in Moscow, IAEA has issued the best possible medical engineers and health physician experts in aid of health management for the victim (IAEA 2001). During the course of the medical intervention, the patient has shown frequent signs of intoxications and physical distress; however, in less than 10 hours of post-exposure, the patient has already developed multiple organ compromise (Konchalovsky, Baranov, and Kolganov 2005). The death of the overexposed engineer is considered a rare type of fatality due to the instance of severe fast-phased extension of the signs and symptoms. Nevertheless, the accident has evidently involved a breach in the facility protocol and one angle of the incident is the policy implementations and workforce management from lower to higher positions. According to Keyes (2004), the quantity of uranium used and the geometrical shape of the fragment have caused the criticality, which eventually resulted to high levels of neutron energy in the form of heat (147).
Despite of the evident breach in the facility protocols, the responsibility of the incident has been thrown to the Russian Federal ministries, commissions and government bodies in consideration to policy implementation and the safety of facilities. According to IAEA (2001), the Ministry for Atomic Energy has included the (1) guarantee of safety of their federal nuclear installations, and the (2) management of radioactive waste and contaminated areas. Hence, the incident is considered a least expected outcome from the facility, and possibly an indicator of management flaws.
- Aims of the Study
In this study, the primary focus revolves in the accident that occurred to a senior engineer at Sarov, Nuclear facility. The study explores the different angles of the (1) potential causes of the incident, (2) the course of action to prevent the occurrence of the accident, (3) the effects of the exposure to the victim, and (4) the mechanisms and physiological explanations involved. The study is limited in covering the criticality accident that occurred only in Sarov, Nuclear Centre, Russian Federation.
- Problem Statement
During the course of the study, the questions that need to be answered are the following:
- What were the possible etiologies that influenced or contributed to the occurrence of the incident?
- Why did the technician die despite of the immediate and prompt medical attention given by different medical and nursing teams?
- How did the intoxication of the substance progressed in the body of the victim and what were the identified signs and symptoms?
- Significance of the Study
Significantly, the outcome of the paper aims to determine the appropriate and applicable lessons learned from the incident. Deriving in these from mentioned contributing factors, these lessons can potentially provide possible preventive and/or promotive interventions that might minimize the possibility of repeating the similar incident.
Discussion
Analysis and Description of the Accident
In June 171997, around 9:30 in the morning, the senior technician, who was 41 is 41 year old male with the height of 1.80 m and weight of 81 kg, had started monitoring and assembling the previously functioning facility containing highly enriched uranium core and copper reflector. According to Musolino (2001), reports had provided significant records of the technician’s experiences and previous criticality experiments. The assembly of the critical facility was rather considered basic compared to the previous tasks of the technician (IAEA 2001). However, Vargo (1999) cited that the technician, at that moment, was alone in handling such procedure. According to IAEA (2001), the preparation and implementation of the senior technician was a considerable violation of the facility protocol, and due to the extent of his years of experience, the technician should have been well versed to the possible dangers of such action.
In fact, IAEA (2001) had mentioned that, “Regulatory and technical documents of the Russian Federation Ministry for Atomic Energy specify precisely the procedural and management requirements for work with critical assemblies. They require that this type of work be conducted only by groups of trained technicians, with each person being responsible for strictly defined actions within a sequence of operations which are closely supervised (10).” However, Vargo (1999) had mentioned the possibility of poor protocol implementation within Russian nuclear facilities considering the presence of fourteen discovered criticality incidents, which occurred from 1953 to 1997. In addition, Vargo (1999) had also pointed out the compromised facility designs of the area that further risks the safety of the technical groups.
By 10:30 in the morning, the victim was about to initiate the criticality assembly procedure; however, during the process, around 10:50, the component from the upper reflector slipped from the technician’s rubber gloved hand and fell on the lower portion of the assembly (IAEA 2001). According to the IAEA International Labour Office (1999), wearing of gloves had always been part of the fundamental protocols of the facility in order to prevent possible contamination on the components of the assembly. However, due to the incident and the compromise of the uranium core to the thermal components in the site, the point of criticality was over-breached producing a flash of light and a form of heat wave. Due to the unexpected initiation and production of force, the lower part of the criticality assembly was pushed back down to the bottom of the stand resulting further instability (IAEA 2001). The event had evidently caused the uncontrollable chain reaction. Principally, the free neutrons produced during the fission end up by either (1) being lost to the external environment, or (2) being absorbed via non-fissile material (Keyes 2004).
Around 10:52, the victim realized the occurrence of criticality accident and considered it as a fatal event. With haste, the victim left the experimental area and closed the sliding doors connecting the other rooms. Furthermore, he informed his supervisors, immediate heads and colleagues regarding the incident and the fatality risk of his exposure (IAEA 2001). At exactly 10:59, initial assessment of his exposed body had been conducted. In the first few minutes of post-exposure and the victim’s report, he was still manifesting signs of stability evident in his cognitive cohesion, consciousness and comprehension. Meanwhile, the concerned heads alerted the radiation protection personnel about the incident.
Direct measurements of neutron induced gamma radiation emitted by his body’s radionuclides had indicated exposure dose of approximately 10 Gy. According to the National Commission on Radiological Protection (NCRP; 1996), the standard amount of exposure requiring immediate medical attention is only 2 Gy; however, based from the initial radiological assessment, the patient had already accumulated 10 Gy, which implied excessive intoxication. Upon determining the extent of exposure, the head of the area ordered immediate evacuation of the building, which occurred from 11:00 to 11:09 (IAEA 2001). On the other hand, the victim’s personal dosimeter was subjected for prompt assessment, while the victim was sent to the local hospital at 11:20, specifically at the occupational medical service for immediate medical action.
From 10:53 to 11:25, the initial response of the operators and radiation protection personnel were the following:
Table 1.1: Initial Response of the Operators and Radiation Protection Personnel: Safety Perspective (June 17, 1997) | |
TIME FRAME | RESPONSE |
10:53 – 10:55 |
|
10:56 – 10:59 |
|
11:00 – 11:09 |
|
11:10 – 11:20 |
|
Note: For comparison of initial response appropriateness in accordance to the Russian Federal Nuclear Centre Emergency Plan (IAEA 1998); (IAEA 2001 12)
Around 13:00, the External Radiation Survey and Radiation Protection Personnel had reported the absence of airborne or surface contamination within the experimental hall (IAEA 2001). According to Putman and Minnema (2005), aside from the fatality exposure, staff assessments had concluded two exposed staff amounting to 1 Gy, while another five had obtained >0.01 Sv. Meanwhile, during the process of assessment, the monitoring unit had located the persistent state of criticality on the assembly emitting significant contents of neutron and gamma radiation (IAEA 2001). Furthermore, neutron flux had been detected in almost all parts of the assembly; however, the influx monitor had indicated the stability of this activity (Musolino 2001). Due to the technicalities of placement and control configuration of the critical assembly, the periscope revealed the complexity of terminating the chain reaction occurring (Gubanov 1999). Hence, it was necessary to remove the remote part of the critical assemble to alter the configured setting in some ways (IAEA 2001). A remotely operated overhead crane present in the experimental area was the only alternative method at that point; however, any movement close to the assembly would trigger an increased reactivity, which might induce extensive heat and radiation generation.
Hence, it was extremely important to avoid any possible positioning or movement of nearby object in the process of dismantling the assembly (IAEA 2001). According to Musolino (2001) and IAEA (2001) reports, the assembly was procedurally removed by (1) using robotic instrument constructed by the Bauman University of Computer Engineering in Moscow, (2) robot manipulator with thin walled conical vacuum suction device (connected to the crane) that stopped the chain reaction and neutron production, and (3) the remote-operated overhead crane that dismantled the entire critical assembly. The entire process was completed only around 1:20 of June 24th 1997 (IAEA 2001).
Criticality Analysis
During the accident, Russian Federation nuclear facilities had conducted intense screening and monitoring, particularly in three perspectives, to better grasp the extent of criticality. These included (1) the staffs present within the site perimeter, (2) the fatally exposed victim, and (3) the criticality assembly and its holding area. According to Turner (2007), the absorption of a neutron gives rise to the radioactive 24Na that possesses a half-life of 15 hours and emits two gamma rays, which contain energies of 2.75 MeV and 1.37 MeV per disintegration (225). According to Khodalev, Tarasova and Zhitnik (1998), the initial exposure of the victim to neutron fission and other components of criticality were not uniform. The capture cross section of the thermal-neutron section bombarding the uranium atom is specific to the value of 0.534 barn (Turner 2007). In fact, higher doses were expected to be found in the upper extremities, particularly in the exposed gloved hand as well as the upper arm.
In response to this thought, the Russian Federal Nuclear Centre’s Monitoring Team had conducted an immediate calculation directed to the exposure. Using a combined beta-gamma neutron personal dosimeter (type GNEIS), the assessment arrived at 10 Gy measurement (IAEA 2001). Since, the advancement of dose distribution was focused in the upper extremities, rhodium (103Rh) neutron activation detection and Aluminum filter in IKS TLD were used to initially measure the neutron activity, which consequently indicated fast neutron dose expansion of 50 Gy. Considering the fast neutron activity, estimated accuracy of dose distribution had also been detected via neptunium fission track dosimeter (IAEA 2001). According to Ziver, Pain and Eaton (2003), the fact that worker’s hands were nearer to the uranium metal sphere explains why neutron influence was larger compared to all other pixels. Ray tracing method was used to calculate dose distribution of neutron and gamma fluences.
As seen in Fig. 1, the energy was the lower torso is lower compared to the areas of the hand. Despite of continuous increase, the pattern remained the same until energy level reached its peak point (Ziver, Pain and Eaton 2003). Meanwhile, to support the phase of neutron dissemination, the neptunium fission track dosimeter indicated an estimated dose of 45+ Gy, which correlated to the neutron flux on the center of the chest (dosimeter: (1.8 + 0.2) x 1012 cm -2). In addition, calculated from the average results of TLD elements and the detector, the results of gamma dose measurement had been 3.5 + 0.3 Gy (IAEA 2001). From these quantitative results, the exposed hands of the technician (10 cm distance from the criticality assembly) were expected to contain 250 Gy. Meanwhile, the exposed body of the individual should contain neutron distribution dose of at least 8 to 11 Gy (IAEA 2001). Using the Monte Carlo method to calculate the spectra of neutrons and gamma radiation and the spectrum of the neutron activity, the suggested total dose that would be available in the hands of the victim was around 800 to 2000 Gy. Although, such results still varied due to the distance component (1 to 5 cm) between technician’s hand and the assembly that were used in the calculation. According to IAEA (2001), the final estimates were 1700 + 170 Gy and about 120 Gy for the neutron and gamma components respectively (20).
On the other hand, according to the calculations of Ziver, Pain and Eaton (2003), the expected neutron and gamma doses should amount to (1) 40.2 Gy (neutron) and 0.6 Gy (gamma) in the hands (dose ratio: 67 Gy), and (2) 30.9 Gy (neutron) and 0.3 Gy (gamma) in the upper chest (dose ratio: 62 Gy). These two results were the highest dose distributions of both gamma and neutron, particularly in the hands ands upper body skin. Dose distribution was already expected to increase, especially for the part exposed nearest to the Uranium core. However, after the patient’s arrival in Moscow’s Institute of Biophysics of the Ministry of Health, the test results revealed by the radiation monitor showed the non-uniform distribution of neutron and gamma activity even within the internal organs of the patient’s body (Wheatley 2001; Trompier, Battaglini and Tikunov 2008). According to IAEA (2001), does distributions in these parts were only confirmed after the first dosimetry results in June 18, 1997, which provided the basis of approximation for absorbed gamma activity and that is between 200 and 300 Gy.
Dose distributions within the internal body were indicated specifically in the table 2 below. Neutron dose distribution was already expected to be higher and the approximation in the trends of its activity was presented. Considering the newly formed fragment of 23Na as a normal constituent of blood compound (Turner 2007; IAEA 2001), the tendency of activating blood sodium can be used as a dosimetric tool when persons are exposed to relatively high doses of neutrons (Turner 2007 225). In the process, activity concentrations of 290 Bq/mL and 260 Bq/mL were used in accordance to the mean whole body neutron dose of 14 Gy. Such procedure was used in order to determine the dose distribution of these substances within the body of the technician.
Table 2: Calculated Dose Distribution on Internal Organs 10 Hours after Exposure | |||
Organ Presented | Neutron Dose (Gy) | Gamma Dose (Gy) | Dose Ratio |
Lungs | 23.4 | 0.5 | 47 |
Stomach | 22.4 | 0.4 | 56 |
Colon | 14.5 | 0.3 | 48 |
Liver | 18.0 | 0.3 | 60 |
Note: Ziver, Pain and Eaton 2003; Dose distribution within the internal organs are illustrated to reveal the difference and the most crucial parts affected by the exposure
Discussion of the Accident
From the results of dose distribution analysis in all exposed body parts of the technician, medical approximation had already considered the possibilities of (1) multiple organ failure, (2) extra-pyramidal effects, (3) high dose variations, and (4) severe expansion of neutron and gamma fluences. According to Konchalovsky, Baranov and Kolganov (2005), the death of the technician was considered due to the severe complications of multi-organ failure brought by acute radiation syndrome. An exposure of 10 Gy was already considered lethal; however, with exposure increased to 200 to 300 Gy, the tendencies of unknown manifestations were highly increased. Konchalovsky, Baranov and Kolganov’s (2005) study found that with an amount total dose more than 20 Gy, (1) microcirculation and leakage syndrome, (2) severe multi-organ failure, (3) encephalopathy, and (4) various iatrogenic problems were most likely to occur (Akashi 2005).
During the initial hours of the victim’s exposure, the victim was still showing signs of consciousness and appropriate comprehension. From the time of exposure, the first noted complications were (1) extensive vomiting with frequency of 4 times per hour and increasing frequency every two hours, (2) fatigue, (3) nausea and (4) dizziness (OECD 2004 218). Meanwhile, the initial interventions provided were (1) administration of anti-emetic drugs (metoclopromide hydrochloride and atropine), (2) infusion of detoxifying drugs (polyvidone 6%, prednisolone 90 mg and glucose 5%), and (3) bodily assessments. Within 3 to 4 hours, the patient’s overall condition had worsened manifesting signs of (1) severe fatigue, (2) headache, (3) pallor, (4) shivering, (5) confusion, (6) excessive perspiration, (7) the occurrence of invasive edema, especially in the hands, and (8) manifestation of invasive erythema in the hands as well (IAEA 2001). According to Chiba, Saito and Ogwa (2002), acute radiation syndrome contributes immediate circulatory inhibition and systemic depression.
n fact, Hirama and Akashi (2005) had stated that upon exposure to radiation, systemic inhibition occurs mostly on soft areas of the body, such as internal organs, smooth muscles and bone marrow. With the intensive alteration in functioning of these parts, hypotension (from the patient’s normal 90/50 down to 80/60), lympophenia, hyperaemia (excessive blood flow) in the chest area, and radiating and intolerable pain in the exposed hands had all occurred during the first 12-24 hours of the incident. After the transfer of the victim from local to specialized hospital care, various complications had been detected, particularly (1) chromosomal aberrations on iliac and thoracic region (Voisin, Roy and Hone et al. 2004; Pyatkin, Nugis and Chirkov 1991)), (2) dropped in circulation, (3) Oliguria, (4) infection, (5) hyperthermia, (6) thrombosis, and (7) necrosis (Ricks, Berger and Ohara 2002).
In order to intervene and correct these manifestations, the medical team provided (1) IVF infusion of 200 ml/h down to 100 ml/h due to progression of Oliguria, (2) irrigation of both hands by Lioxazol, Acyclovir, Ketoconazole and Ciprofloxacin, (3) continuous sodium heparin perfusion and (4) administration of Aprotinin. Unfortunately, due to unexpected bodily reaction to fluid administration and fluid retention drugs, vascular damage occuured, hypocalcaemia, acidosis, lung edema, hypoxemia, and lung compression due to peritoneal fluid excess occurred. Furthermore, severe hypotension, bradycardia and heart failure also occurred, and on June 20, 1997 at around 3:20, the victim died despite of 66 hours of prompt medical action (IAEA 2001).
Lessons Learned
There are two points of considerations on the lessons learned from this incident, particularly (1) the implementation of safety regulations, and (2) the question on appropriate medical prognosis and decision. Considering the policy violation done by the senior technician, the policy being implemented must be tolerating in a sense and probably not being strictly implemented, which consequently supported the victim’s intention of risking himself in the process. Meanwhile, at 15:30, the monitoring and assessment team had reported that the victim did not actually absorb high amounts of the substance; however, with the advancing signs and symptoms, observations of induced gamma activity and the description of the accident, poor prognosis was also cited as one of faults in the intervention. After 10 hours of post-incident passed, the medical team had decided to send the case to the nearest specialized hospital in Clinical Department Institute of Biophysics in Ministry of Health (20:50). Under these conditions, it is appropriate to say that a policy should be implemented strictly especially for extremely risky tasks, and should not exempt anyone on the basis of any reason. Meanwhile, if such accident occurs, it is also essential to revise the intervention protocol of sending the victim immediately to the specialized hospital regardless of initial assessment.
Conclusion
In the study, the Sarov accident resulting to the death of a nuclear facility senior technician has been analyzed as the primary subject. The accident was due to the victim’s exposure to a criticality assembly’s uranium core after his gloved hand slipped during the process of his actions. He directed the concern to his colleagues and other heads immediately, and they acknowledged the incident as the highest priority emergency at that point. The victim was monitored by various procedure and found that the exposure levels absorbed by his body was more than it could normally handle (10 Gy). In response to the incident, the medical team sent the client to the nearest local hospital for immediate treatment. However, the case and neutron activity had climbed the dose of 200 to 300 Gy in less than 10 hours. The victim immediately experienced severity in the manifesting signs and symptoms.
On June 20, 1997, the victim died due to severe drop in blood pressure and heart failure. To answer the questions of the paper, the possible etiologies identified in the process of the accident were (1) the fault in the implementation of the policy, and (2) violation of rules and safety protocols of the facility. Meanwhile, wrong prognosis was considered as the primary reason why the client died despite of medical attention. In addition, the end dose content in the body of the patient was excessively more than it could handle. The neutron activity spread from 10 Gy to its final count of 800 to 2000 Gy, which actually bombarded all areas of the victim’s body. Major signs and symptoms identified were severe hypotension, bradycardia, invasive edema, and thoracic and pleural compression, which eventually resulted to heart failure causing the victim’s death.
Bibliography
Akashi, M. “Role of infection and bleeding in multiple organ involvement and failure.” British Journal of Radiology 10.12 (June 2005): 69-74.
Chiba, S, A Saito, and S Ogwa et al.. “Transplantation for accidental acute high-dose total body neutron- and bold gamma- y radiation exposure.” Bone Marrow Transplantation 29.11 (June 2002): 935-939.
Gubanov, V A. “Russian Viewpoint on the Safety of Nuclear Materials.” Ministry of Atomic Energy 11.3 (June 1999): 24-26.
Hirama, T, and M Akashi. “Multi-organ involvement in the patient who survived the Tokai-mura criticality accident.” British Journal of Radiology 27 (June 2005): 17-20.
IAEA – World Health Organization, . Planning the Medical Response to Radiological Accidents, Safety Reports Series No. 4. Vienna: IAEA, 1998.
IAEA. International Energy Agency: International Labour Office – Occupational Radiation Protection, Safety Standards Series No. RS-G-1.1. Vienna: IAEA, 1999.
International Atomic Energy Associat, . “The Criticality Accident in Sarov.” The Criticality Accident in Sarov. 1 IAEAInternational Atomic Energy Association (IAEA) . 1 Feb. 2001 <http://www-pub.iaea.org/MTCD/publications/PDF/Pub1106_scr.pdf>.
Keyes, Daniel C. Medical Response to Terrorism: Preparedness and Clinical Practice. New York, U.S.A: Lippincott Williams & Wilkins, 2004.
Khodalev, G F., E Y. Tarasova, and A K. Zhitnik. “Radiation dose received by a researcher in a critical assembly accident at Russian Federation Nuclear Center/All-Russian Scientific-Tesearch Institute of experimental physics.” Journal of Atomic Energy 85.2 (Aug. 1998): 578-582.
Konchalovsky, M V., A E. Baranov, and A V. Kolganov. “Multiple organ involvement and failure: selected Russian radiation accident cases re-visited.” British Journal of Radiology 27.1 (2005): 26-29.
Musolino, Stephen B. “Radiation dose received by a researcher in a critical assembly accident at Russian Federation Nuclear Center/All-Russian Scientific-Tesearch Institute of experimental physics.” Journal of Atomic Energy 85.2 (Aug. 1998): 578-582.
Musolino, Stephen B. “The Criticality Accident in Sarov.” Health Physics 81.5 (Nov. 2001): 593-608.
National Commission on Radiological Safety Standards. Russian Radiation Safety Standards. Moscow: NCRP, 1996.
Organization for Economic Co-Operati, . Computing Radiation Dosimetry: CRD 2002. New York, U.S,A: OECD Publishing, 2004.
Putman, Valerie L., and Douglas M. Minnema. “First Responders and Criticality Accidents.” Transactions of the American Nuclear Society 93.5 (Nov. 2005): 210-212.
Pyatkin, E K., V Y. Nugis, and A A. Chirkov. “Analysis of chromosome aberrations for evaluation of severity of the bone marrow syndrome of radiation disease.” Radiation Protection Dosimetry 36.10 (Oct. 1991): 21–26.
Ricks, Robert C., Marry Berger, and Fredrick M. Ohara. The Medical Basis for Radiation-accident Preparedness. New York, U.S,A: Taylor & Francis, 2002.
Trompier, F, P Battaglini, and D Tikunov. “Dosimetric response of human bone tissue to photons and fission neutrons.” Journal of Radiation Measurement 43.2 (June 2008): 37-840 .
Turner, James E. Atoms, Radiation, and Radiation Protection. London, U.K: Wiley-VCH, 2007.
Vargo, George J. “A Brief History of Nuclear Criticality Accidents in Russia-1953-1997.” Journal of Health Physics 77.5 (Nov. 1999): 505-511.
Voisin, P, L Roy, and P L. Hone. “Criticality accident dosimetry by chromosomal analysis.” Radiation Protection Dosimetry 110.1 (Jan. 2004): 443-447.
Wheatley, John. “IAEA’s Radiation Events Database.” Radiological Protection of Patients in Diagnostic and Care 3.1 (Aug. 2001): 1-7.
Ziver, A K., C C. Pain, and M D. Eaton et al.. “Comparative Neutron and Gamma-ray Dosimetry of JCO and SAROV Accidents Using FETCH.” JAERI Conference 25.1 (2003): 808-813.