Substituents on Aromatic Aldehydes and How They Affect E/Z Selectivity in the Wittig Reaction
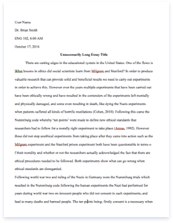
- Pages: 20
- Word count: 4760
- Category: Chemistry
A limited time offer! Get a custom sample essay written according to your requirements urgent 3h delivery guaranteed
Order NowThe Wittig Reaction is a nucleophilic addition in which an alkene is formed as a product. Both the E and Z isomers of the alkene result. Substituents on the aromatic aldehyde affect the E/Z ratio of products that form. In this experiment, a nitro group was used as the substituent in the ortho, meta and para positions, with benzaldehyde as the control. Each of the four aldehydes reacted with (carbethoxymethylene) triphenylphosphorane to produce ethyl cinnamate, ethyl-3-(2-nitrophenyl)acrylate, ethyl-3-(3-nitrophenyl)acrylate, and ethyl-3-(4-nitrophenyl)acrylate.
The prediction was the closer the substituent was to the aldehyde, the greater the ratio of E/Z isomers; benzaldehyde was predicted to result in the most similar ratio of E/Z isomers. The ratios of E/Z isomers were determined by using the NMR spectra obtained. A percent conversion was also obtained. Ethyl-3-(2-nitrophenyl)acrylate had a ratio of 9.03:1, ethyl-3-(3-nitrophenyl)acrylate had a ratio of 5.2:1, ethyl-3-(4-nitrophenyl)acrylate had a ratio of 1:1 and ethyl cinnamate had a ratio of 9.3:1. The results for the compounds except ethyl cinnamate supported the hypothesis that the ratio would be closer to 1:1 as the substituent moved further from the carbonyl.
Introduction:The purpose of this experiment is to determine how a nitro group on benzaldehyde affects the stereoisomer formation of the alkene formed in a Wittig Reaction. The nitro group is substituted in the ortho, meta, and para positions, and benzaldehyde serves as a control. The ylide used is (carbethoxymethylene) triphenylphosphorane. Through the utilization of NMR spectroscopy, the products of the reactions will be analyzed to gain a ratio of E /Z isomers.
Reaction Equations:Ethyl cinnamate from BenzaldehydeEthyl-3-(2-nirophenyl)acrylate from O-nitrobenzaldehydeEthyl-3-(3-nitrophenyl)acrylate from M-nitrobenzaldehydeEthyl-3-(4-nitrophenyl)acrylate from P-nitrobenzaldehydeIn 1954, Georg Wittig, a German chemist, discovered the Wittig Reaction. In 1979, Wittig won a Nobel Prize in Chemistry due to the Wittig Reaction’s synthetic potential to form a variety of different alkenes. Today, this reaction is used to synthetically alkylate many different compounds that may otherwise be impossible to complete due to steric hindrance.
The Wittig Reaction is a specific type of nucleophilic addition to carbonyls to form an alkene. This reaction is very important and powerful because a C-C bond is formed and numerous combinations of starting materials produce an extremely diverse number of products. The starting materials for a Wittig Reaction are a compound with a carbonyl group and a phosphorus ylide. The carbon of the carbonyl is a strong electrophile because the oxygen pulls electron density away from the carbon, due to the inductive effect. A phosphorus ylide is a neutral compound that has a separation of formal charges. The atom in the ylide that contains the negative charge is the nucleophile that attacks the electrophilic carbon of the carbonyl. The products of the reaction are an alkene and triphenylphosphine oxide.
An ylide is formed when triphenylphosphate and an alkyl halide react with a strong base. SN2 chemistry is performed, and forms a phosphonium salt and a halide. The base deprotonates the phosphonium salt to form the phosphonium ylide, which is also called the Wittig reagent. The ylide has a positive charge on the phosphorus and a negative charge on the carbon, which makes the compound strongly nucleophilic at the carbon.
Water is used as the solvent to make the reaction “green” so the waste and byproducts are not as harsh on the environment. This is a good substitute for the usual solvent dichloromethane, which is carcinogenic. “Greener” techniques in a laboratory setting are important because they reduce damage to the environment caused by using harsh chemicals. These chemicals cause serious problems when released in excess into the air or water.
The E/Z stereoisomer can form as the alkene product in this reaction. The E isomer is more stable due to sterics and is the major product in many reactions. The isomer that forms depends on the substituents on the aldehyde and the substituent’s connectivity. Benzaldehyde has no substituents to cause steric strain, therefore the smallest ratio of E/Z alkenes will form. The closer the nitro group is to the aldehyde, the larger the ratio of E/ZÂ alkenes. The further away the nitro group is substituted from the aldehyde, the smaller the ratio of E/Z alkenes.
Experimental Section:Ethyl CinnamateTo a disposable screw capped vial, 0.11 g of benzaldehyde, 4 mL of water and a magnetic stir bar were added, along with 0.38 g of (carbethoxymethylene) triphenylphosphane. The mixture was stirred for 45 minutes. The solid in the vial clumped together around the stir bar, so vigorous shaking was used to dislodge the stir bar every few minutes. The solid in the vial was dough like. Three grams of anhydrous sodium sulfate was added to a 50 mL Erlenmeyer flask. Five extractions were performed with 2 mL aliquots of 1:1 ether/hexane. After each extraction, the organic layer was identified, extracted out of the vial using a Pasteur pipet, and placed into the Erlenmeyer flask which contained the anhydrous sodium sulfate. When the last extraction was performed, the water layer was removed and put into a separate beaker. The organic layer was removed last and placed into the Erlenmeyer flask. The solution was dried over sodium sulfate for five minutes.
A clean, dry filter flask was obtained, and a vacuum filter apparatus was set up. The solution was then vacuum filtered. The Erlenmeyer flask was rinsed with 2 mL of 1:1 ether/hexane solution, and the contents were emptied into the vacuum filter. The solution was filtered for three minutes. The funnel was replaced with a rubber stopper, and the contents in the flask remained under vacuum pressure to remove all the solvent. After the solvent was removed, the products remained under vacuum for five minutes. The product was an oily substance that clung to the side of the flask.
An NMR sample was prepared by adding 10 drops of chloroform to the product, and was swirled to mix. Five drops of this mixture was added to the NMR tube along with additional chloroform. An NMR spectroscopy was performed on the product. The spectrum was analyzed to determine the E:Z ratio of the products formed and the percent conversion. The percent conversion was determined by analysis of the NMR data around 10 ppm, where aldehyde hydrogens would be present. The spectral data for the product is tabulated in Table 1. Refer to figures 1 through 3 for the NMR spectra.
Ethyl-3-(2-nitrophenyl)acrylateTo a disposable screw capped vial, 0.15 g of o-nitrobenzaldehyde, 4 mL of water and a magnetic stir bar were added, along with 0.38 g of (carbethoxymethylene) triphenylphosphane. The mixture was stirred for 45 minutes. The solid in the vial clumped together around the stir bar. Vigorous shaking was used to dislodge the stir bar every few minutes. The solid in the vial was dough like. Three grams of anhydrous sodium sulfate was added to a 50 mL Erlenmeyer flask. Five extractions were performed with 2 mL aliquots of 1:1 ether/hexane. The organic layer was extracted out of the vial using a Pasteur pipet and placed into the Erlenmeyer flask which contained the anhydrous sodium sulfate. When the last extraction was performed, the water layer was removed and put into a separate beaker. The organic layer was removed last and placed into the Erlenmeyer flask. The solution was dried with sodium sulfate for five minutes.
A clean, dry filter flask was obtained and a vacuum filter apparatus was set up. The solution was vacuum. The Erlenmeyer flask was rinsed with 2 mL of 1:1 ether/hexane solution and the contents were emptied into the vacuum filter. The solution was filtered for three minutes. The funnel was replaced with a rubber stopper, and the contents in the flask remained under vacuum pressure to remove all solvent. The products remained under vacuum for five minutes. The product was an oily substance that clung to the side of the flask.
An NMR sample was prepared by adding 10 drops of chloroform to the product, and was swirled to mix. Five drops of this mixture was added to the NMR tube along with additional chloroform. An NMR spectroscopy was performed on the product. The spectrum was analyzed to determine the E/Z ratio of the products formed and the percent conversion. The percent conversion was determined by analysis of the NMR data around 10 ppm, where aldehyde hydrogens would be present. The spectral data for the product is tabulated in Table 2. Refer to figures 4 through 6 for the NMR spectra.
Ethyl-3-(3-nitrophenyl)acrylateTo a disposable screw capped vial, 0.15 g of m-nitrobenzaldehyde, 4 mL of water and a magnetic stir bar were added, along with 0.38 g of (carbethoxymethylene) triphenylphosphane. The mixture was stirred for 45 minutes. The solid in the vial clumped together around the stir bar. Vigorous shaking was used to dislodge the stir bar every few minutes. About three grams of anhydrous sodium sulfate was added to a 50 mL Erlenmeyer flask. Five extractions were performed with 2 mL aliquots of 1:1 ether/hexane. The organic layer was extracted out of the vial using a Pasteur pipet and placed into the Erlenmeyer flask which contained the anhydrous sodium sulfate. When the last extraction was performed, the water layer was removed and put into a separate beaker. The organic layer was removed last and placed into the Erlenmeyer flask. Additional sodium sulfate was added until all water was removed from solution. The solution was dried with sodium sulfate for five minutes.
A clean, dry filter flask was obtained, and a vacuum filter apparatus was set up. The solution was vacuum filtered. The Erlenmeyer flask was rinsed with 2 mL of 1:1 ether/hexane solution, and the contents were emptied into the vacuum filter. The solution was filtered for three minutes. The funnel was replaced with a rubber stopper, and the contents in the flask remained under vacuum pressure to remove all solvent. The products remained under vacuum for five minutes. The product was a solid.
An NMR sample was prepared by adding 10 drops of chloroform to the product, and was swirled to mix. Five drops of this mixture was added to the NMR tube along with additional chloroform. An NMR spectroscopy was performed on the product. The spectrum was analyzed to determine the E/Z ratio of the products formed and the percent conversion. The percent conversion was determined by analysis of the NMR data around 10 ppm, where aldehyde hydrogens would be present. The spectral data for the product is tabulated in Table 3. Refer to figures 7 through 9 for the NMR spectra.
Ethyl-3-(4-nitrophenyl)acrylateTo a disposable screw capped vial, 0.15 g of p-nitrobenzaldehyde, 4 mL of water and a magnetic stir bar are added, along with 0.38 g of (carbethoxymethylene) triphenylphosphane. The mixture was stirred for 45 minutes. The solid in the vial clumped together around the stir bar, so vigorous shaking was used to dislodge the stir bar every few minutes. The solid in the vial was dough like. Three grams of anhydrous sodium sulfate was added to a 50 mL Erlenmeyer flask. Five extractions were performed with 2 mL aliquots of 1:1 ether/hexane. After each extraction, the organic layer was identified, extracted out of the vial using a Pasteur pipet, and placed into the Erlenmeyer flask containing the anhydrous sodium sulfate. When the last extraction was performed, the water layer was removed and put into a separate beaker. The organic layer was removed last and placed into the Erlenmeyer flask. Additional sodium sulfate was added until all of the water was removed from solution. The solution was dried over sodium sulfate for five minutes.
A clean, dry filter flask was obtained, and a vacuum filter apparatus was set up. The solution was then vacuum filtered. The Erlenmeyer flask was rinsed with 2 mL of 1:1 ether/hexane solution and the contents were emptied into the vacuum filter. The solution was filtered for three minutes, then the funnel was replaced with a rubber stopper, and the contents in the flask remained under vacuum pressure to remove all the solvent. After the solvent was removed, the products remained under vacuum for five minutes. The product was an oily substance that clung to the side of the flask.
An NMR sample was prepared by adding 10 drops of chloroform to the product, and was swirled to mix. Five drops of this mixture was added to the NMR tube along with additional chloroform. An NMR spectroscopy was performed on the product. The spectrum was analyzed to determine the E:Z ratio of the products formed and the percent conversion. The percent conversion was determined by analysis of the NMR data around 10 ppm, where aldehyde hydrogens would be present. The spectral data for the product is tabulated in Table 4. Refer to figures 10 through 12 for the NMR spectra.
Discussion:Ethyl CinnamateThe NMR spectrum of ethyl cinnamate was analyzed to find characteristic peaks. The alkene protons were found between 5.5 and 7.5 ppm. The peaks at 6.45 and 6.9 ppm were doublets which corresponded to the protons of the alkene. The coupling constant, the distance between the two peaks of a doublet, was measured for both of the peaks to determine which doublet represented the E and Z isomers. A coupling constant is larger for E isomers than Z isomers. The peak at 6.45 ppm had a coupling constant of 0.5 cm, and peaks at 6.9 ppm and 5.9 ppm had a coupling constant of 0.4 cm. Therefore, the E isomer was the peak at 6.45 ppm and the Z isomer was the peak at 6.4 ppm. Similar coupling constants in other peaks determined which isomer the peak represented.
The chemical shift of 1.25 ppm corresponded with impurities. A quartet found at 4.24 ppm corresponded with the -CH2 group on the Z isomer, and the quartet found at 4.26 ppm represented the -CH2 group on the E isomer. The peaks at 5.9 and 6.45 ppm correspond with the hydrogens on the alkene for both the Z and E isomer. These peaks were key in the determination of the different isomers present. The doublet with a chemical shift of 5.9 ppm also corresponded to hydrogens on the Z alkene because the integration and coupling constant were identical to the shift of 5.9 ppm. The second E isomer doublet was found in the aromatic region, so the integration could not be determined. The set of multiplets from 7.25 to 7.70 ppm represented aromatic hydrogens.
The ratio of E/Z isomers was obtained when the two doublets in the 5.5-7.5 ppm range were compared. After identifying which doublet corresponded to which isomer, the ratio was obtained by dividing the integration of the E isomer by the integration of the Z isomer. The integrations were 0.56 ppm and 0.06 ppm, so the ratio was 9.3:1 E to Z isomers. This differs from the predicted value of a small ratio. This difference was caused by the lack of NO2 on the aldehyde, so steric hinderance was absent. The percent conversion for the reaction was calculated by identifying traces of unreacted starting material. If aldehyde was present the peaks would have appeared at 10 ppm. However, no peaks were present at this location, so the conversion was assumed to be greater than 99.5%.
Ethyl-3-(2-nitrophenyl)acrylateThe NMR spectrum of ethyl-3-(2-nitrophenyl)acrylate was analyzed to find characteristic peaks. The alkene protons were found between 5.5 and 7.5 ppm. The peaks at 6.1 and 6.4 ppm were doublets which corresponded to the protons of the alkene. The coupling constant, the distance between the two peaks of a doublet, was measured for both of the peaks to determine which doublet represented the E and Z isomers. A coupling constant is larger for E isomers than Z isomers. The peak at 6.1 ppm had a coupling constant of 0.3 cm, while the peak at 6.4 ppm was 0.4 cm. Therefore, the Z isomer is the peak at 6.1 ppm and the E isomer is the peak at 6.4 ppm. Similar coupling constants in other peaks determined which isomer the peak represented.
At 1.1 ppm, a triplet was present, which corresponded to a methyl group on the Z-isomer. The same methyl group on the E-isomer was represented by a triplet at 1.4 ppm. A quartet found at 4.0 ppm corresponded with the -CH2 group on the Z-isomer, and the quartet found at 4.3 ppm represented the -CH2 group on the E-isomer. The peaks at 6.1 and 6.4 ppm corresponded with the hydrogens on the alkene for both the Z and E isomer. These peaks were key in the determination of the different isomers present. The second Z and E isomer doublets were found in the aromatic region, so the integrations could not be determined. The set of multiplets from 7.1 to 7.8 ppm represented that aromatic hydrogens present. Lastly, a doublet at 8.1 ppm corresponded to the alpha hydrogen off of the carbonyl for the E-isomer.
The ratio of E/Z isomers was obtained by comparing the two doublets in the 5.5-7.5 ppm range. After identifying which doublet corresponded to which isomer, the ratio was attained by dividing the integration of the E isomer by the integration of the Z isomer. The integrations were 9.03 ppm and 1.00 ppm, so the ratio was 9.03:1 E to Z isomers. The percent conversion for the reaction was calculated by identifying traces of unreacted starting material. If aldehyde was present, the peaks would have appeared at around 10 ppm. However, no peaks were present at this location, so the conversion was assumed to be greater than 99.5%.
Ethyl-3-(3-nitrophenyl)acrylateThe NMR spectrum of ethyl-3-(2-nitrophenyl)acrylate was analyzed to find characteristic peaks. The alkene protons were found between 5.5 and 7.5 ppm. The peaks at 6.1 and 6.55 ppm were doublets which corresponded to the protons of the alkene. The coupling constant, the distance between the two peaks of a doublet, was measured for both of the peaks to determine which doublet represented the E and Z isomers. A coupling constant is larger for E isomers than Z isomers. The peak at 6.1 ppm had a coupling constant of 0.2 cm, while the peak at 6.4 ppm was 0.3 cm. Therefore, the E isomer is the peak at 6.1 ppm, and the Z isomer is the peak at 6.55 ppm. Similar coupling constants in other peaks determined which isomer the peak represented.
At 1.2 ppm, a triplet was present, which corresponded to a methyl group on the Z-isomer. The same methyl group on the E-isomer was represented by a triplet at 1.25 ppm. The singlet at 1.8 ppm was found to be an impurity in the product. A quartet found at 4.18 ppm corresponded with the CH2 group on the Z isomer, and the quartet found at 4.3 ppm represented the CH2 group on the E-isomer. The peaks at 6.1 and 6.55 ppm corresponded with the hydrogens on the alkene for both the Z and E isomer. These peaks were key in the determination of the different isomers present. The doublet with a chemical shift of 7.0 ppm also corresponded to hydrogens on the Z alkene because the integration and coupling constant are almost identical to the shift of 6.1 ppm. The second E isomer doublet was found in the aromatic region, so the integration could not be determined. The set of multiplets from 7.2 to 8.0 ppm represented that aromatic hydrogens present. Lastly, a doublet at 8.2 ppm corresponded to the alpha hydrogen off of the carbonyl for the E isomer, and a doublet at 8.4 was consistent with alpha hydrogens off of the carbonyl for the Z isomer.
The ratio of E?Z isomers was obtained by comparing the two doublets in the 5.5-7.5 ppm range. After identifying which doublet corresponded to which isomer, the ratio was obtained by dividing the integration of the E isomer by the integration of the Z isomer. The integration of the doublet at 6.1 ppm was 1.00, and the integration at the doublet at 6.55 ppm was 5.20. Therefore, the ratio was 5.2:1 of E to Z isomers. The percent conversion for the reaction was calculated by identifying any traces of unreacted starting material. If aldehyde was present, the peaks would have appeared at around 10 ppm. However, no peaks were present at this location so, the conversion was assumed to be greater than 99.5%.
Ethyl-3-(4-nitrophenyl)acrylateThe NMR spectrum of ethyl-3-(2-nitrophenyl)acrylate was analyzed to find characteristic peaks. The alkene protons were found between 5.5 and 7.5 ppm. The peaks at 6.1 and 6.6 ppm were doublets which corresponded to the protons of the alkene. The coupling constant, the distance between the two peaks of a doublet, was measured for both of the peaks to determine which doublet represented the E and Z isomers. A coupling constant is larger for E isomers than Z isomers. The peak at 6.1 ppm had a coupling constant of 0.25 cm, while the peak at 6.4 ppm was 0.3 cm. Therefore, the Z isomer is the peak at 6.1 ppm and the E isomer is the peak at 6.4 ppm. Similar coupling constants in other peaks determined which isomer the peak represented.
At 1.3 ppm, a triplet was present, which corresponded to a methyl group on the Z isomer. The same methyl group on the E isomer was represented by a triplet at 1.4 ppm. A quartet found at 4.2 ppm corresponded with the -CH2 group on the Z isomer, and the quartet found at 4.3 ppm represented the -CH2 group on the E isomer. The peaks at 6.1 and 6.6 ppm corresponded with the hydrogens on the alkene for both the Z and E isomer. These peaks were key in the determination of the different isomers present. The second Z and E isomer doublets were found in the aromatic region, so the integrations could not be determined. The set of multiplets from 7.2 to 8.4 ppm represented that aromatic hydrogens present. A singlet at 10.1 ppm represented the presence unreacted aldehyde protons. There were two traces of impurities, a singlet at 1.7 ppm and a triplet at 4.9 ppm.
The ratio of E/Z isomers was obtained by comparing the two doublets in the 5.5-7.5 ppm range. After identifying which doublet corresponded to which isomer, the ratio was obtained by dividing the integration of the E isomer by the integration of the Z isomer. The integrations were each 0.09 ppm, so the ratio was 1:1 E to Z isomers. The percent conversion for the reaction was calculated by identifying unreacted starting material. A singlet showed in the NMR around 10 ppm, so the conversion was not greater than 99.5%. The doublet integrations used to identify the ratio of E to Z isomers were summed. The summed integrations of the isomers were divided by the integration of the aldehyde peak. The conversion for this reaction was about 63%.
Mechanism:In the mechanism of this reaction, the ylide, (carbethoxymethylene) triphenylphosphorane is not a salt, so no base is necessary. A lone pair of electrons exists on the carbon adjacent to the phosphorus, so the carbon has a negative formal charge. These electrons attack the most nucleophilic compound present in the reaction. Each reaction used a different aldehyde (benzaldehyde or ortho, meta ,or para-nitrobenzaldehyde) as a nucleophile. The lone pair attacks the carbon of the carbonyl of the aldehyde. This carbon has a partial positive charge because electrons are being pulled through the double bond toward oxygen due to the inductive effect. While carbon is attacked, electrons from the carbon-oxygen double bond move up to the oxygen.
This moving of electrons causes a negative formal charge on oxygen. A lone pair of electrons on the oxygen then attacks the most electrophilic atom in the reaction, the positively charged phosphorus. An unstable four-membered ring, called an oxaphosphetane, forms as an intermediate. The ring breaks when electrons from the carbon-oxygen bond move to form an oxygen-phosphorus double bond. This step of the mechanism generates a triphenylphosphine oxide byproduct. Electrons from the carbon-phosphorus bond move to form a carbon-carbon double bond. The final product is a mixture of the E and Z isomers.
In the procedure of this reaction, 1 mmol of the carbonyl compound was added to deionized water and 1.1 mmol of the ylide. The carbonyl compound is the limiting reagent because a smaller number of moles of the carbonyl are added than the ylide. This method is used to minimize the amount of unreacted starting material. During the extractions, the organic layer was removed with 1:1 ether/hexane solution. The organic layer was the top layer and the aqueous layer was the lower level. This was determined by adding a few drops of water to the solution. The water was added and went through the top organic layer into the aqueous layer. The organic layer was very hydrophobic and was not miscible with water.
On the last extraction, the water layer was removed and placed in a separate beaker. This was done to ensure that no water was transferred into the products and everything extracted was from the organic layer. When the NMR spectra were analyzed, impurities were present in the aromatic region between 6.5 and 8 ppm. This impurity was the byproduct of the Wittig Reaction, triphenylphosphine oxide. This byproduct is found in the aromatic region due to the three aromatic rings. The only reaction that had unreacted starting material was the fourth reaction with p-nitrobenzaldehyde. The other three reactions drove nearly completely to product, because the starting materials were more reactive than p-nitrobenzaldehyde.
In other reactions with the same starting materials, a variety of E/Z ratios were observed. When benzaldehyde reacted with (carbethoxymethylene) triphenylphosphorane in another reaction, a ratio of 15.4:1 was obtained with a conversion of 91%. This data is very similar to what was obtained in the first trial. When o-nitrobenzaldehyde reacted with (carbethoxymethylene) triphenylphosphorane in a separate trial, a ratio of 7.4:1 was obtained with a conversion of greater than 99.5%. In another reaction, m-nitrobenzaldehyde reacted with the same ylide with a ratio of 2.6:1 and a conversion of greater than 99.5%. In the last reaction, p-nitrobenzaldehyde and (carbethoxymethylene) triphenylphosphorane reacted, and a ratio of 2:1 with a conversion of greater than 99.5% was obtained.
This additional information supports the hypothesis for this experiment. The further the substituent from the aldehyde on the ring, the smaller the ratio of E/Z alkenes would be obtained. The closer the substituent to the aldehyde on the ring, the larger the ratio of E/Z alkenes would be obtained. The only trend in this experiment that did not support the hypothesis was the ratio for benzaldehyde. Benzaldehyde was predicted to have the smallest ratio of E/Z alkenes, but had the largest ratio of E/Z. To further study these trends, experiments with more than one substituent could be performed. Two nitro groups could be substituted on the aromatic aldehyde instead of one group to study steric effects in more depth.
This experiment was done in a “green” manner by utilizing water as the solvent instead of the typical dichloromethane. “Green” experiments lessen the effects of waste on the environment. Dichloromethane is very toxic and carcinogenic. Substitution of water as the solvent lessens the harshness of the byproducts. Some noxious compounds used could be traded for safer solvents or methods. Instead of using sodium sulfate to dehydrate the solution, the flask could be allowed to air dry. Rather than using ether/hexane solution for the extractions, an alcohol could be used.
The products of the Wittig Reactions were not pure, do to the presence of extra peaks in the NMR spectra and calculations of percent conversion. One impuritie was triphenylphosphine oxide, which could have entered the products when the organic layer was extracted. Since the extraction was performed with a pipet, human error could have caused the two layers to be mixed. The mixing most likely occurred when the aqueous layer, the bottom layer, was extracted before the organic layer, the top layer, was extracted. The product could also have been contaminated during the preparation of the NMR sample. Chloroform was addedto the product, swirled, then added to an NMR tube. This method of preparation could have caused more errors in the NMR spectra.
References:
Aldrich Advancing Science. Handbook of Fine Chemicals. 2008.
Carey, Francis. Organic Chemistry. Boston: McGraw Hill, 2008.
Interpreting NMR Spectra from your Wittig Reaction and Importing Data into Google Documents. Elizabethtown College Department of Chemistry and Biochemistry. Chemistry 114 Laboratory. 2009.
Science in Action: Alkene Synthesis form a Green Wittig Reaction. Elizabethtown College Department of Chemistry and Biochemistry. Chemistry 114 Laboratory. 2009.