Role and Scope of Ethnomedical Plants in the Development of Antivirals
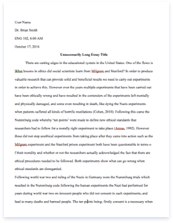
- Pages: 14
- Word count: 3429
- Category: College Example Plantation
A limited time offer! Get a custom sample essay written according to your requirements urgent 3h delivery guaranteed
Order NowSummary Ethnomedicinal plants have been used as source of drugs for almost all diseases, but none are used against viruses probably because there are a very few specific viral targets for natural molecules to interact. Most of the available antiviral drugs often lead to side effects, viral resistance, recurrence and latency. A wide range of ethnomedicinal plants showed strong antiviral activities either by inhibiting replication, or genome synthesis of many viruses. Hence, development of new antivirals from natural source is an alternate approach. This review will discuss some of the promising antivirals of ethnomedicinal plants with proven in vitro and some documented in vivo activities. Keywords: Ethnomedicines; Antivirals, HSV; HIV.
People of all continents have long used poultices and infusions of indigenous plants like cedar wood and cypress oil, juice of licorice, myrrh and poppy for the treatment of coughs and colds to parasitic infections and inflammation1. The clinical virologists are looking into the antiviral plant extracts as (i) the effective life span of antiviral drug is limited; (ii) many viral diseases are intractable to most of the orthodox antivirals, (iii) development of viral resistance, latency and recurrence, and (iv) rapid spread of emerging and reemerging viral diseases like HIV/AIDS, SARS etc. All these spurred intensive investigation into the ethnomedicines, especially for people unable to afford expensive antivirals, and the impressive array of knowledge and wisdom of indigenous people about their generation old medicaments for the development of new or complementary antivirals. 64
Pharmacologyonline 3: 64-72 (2006)
Newsletter
Chattopadhyay
Viral infections can be controlled either by prophylactic or therapeutic measures. As metabolically inert particle viruses require living cells to replicate and hence, it is difficult to design a treatment that attacks the virion without affecting the host. Although there are 37 licensed antivirals, the development of antivirals from natural source is less explored because of a very few specific viral targets for natural molecules to interact with. Fortunately, many viruses have unique features in their structure or replication cycles that can be the potential targets, e.g., the nucleoside analogue acycloguanosine that blocks key enzymes of herpes virus replication2. Due to the amazing structural diversity and broad range of bioactivities many ethnomedicinal plant extracts inhibit several steps of replication or certain cellular factors of many DNA and/or RNA viruses. This review will summarize some of the prospective molecules of ethnomedicinal plants, active against diverse virus families. A list of some potential ethnomedicinal plants and their antiviral principles are presented in Table 1. Major group of antivirals of Ethnomedicinal plants Phenolics: Phenolics are the simplest bioactive phenylpropanes with wide range of antiviral activities. The oligophenols of Peruvian folklore Stylogne cauliflora inhibit hepatitis C virus (HCV) non-structural serine protease3, while polyphenols of Agrimonia pilosa and Punica granatum had anti-herpes simplex virus (HSV) type 1 activity; but the polyphenols of Blumea laciniata and Scutellaria indica inhibit respiratory syncytial virus (RSV) with IC5012.5-32 µg/ml4. The caffeic acid, chlorogenic acid and rosmarinic acid derivatives of Plantago major, an ethnomedicine of Asia, inactivate HSV-1 and HSV-25, varicella zoster virus (VZV), pseudorabies virus (PRV) and influenza viruses (IV)4, that can be developed as antiherpes agent. The phenolics inactivate cell surface proteins, prevent viral adsorption, inhibit viral reverse transcriptase (RTase) and RNA polymerase, and the
structure activity study revealed that the sites and number of OH- on phenols are responsible for their antiviral activity. The proanthocyanidin A1 had remarkable anti-herpes activity that block HSV2 attachment and penetration6, while procyanidin C1 inhibit HIV-17. The anti-HSV activity of epicatechin dimers is due to ortho-trihydroxyl groups and the double interflavan linkages5, while xanthohumol of Humulus lupulus might serve as a lead for anti-HSV agent7. The bis-catechol l-chicoric acid, a dicaffeoyltartaric acid (DCTA), is the most active inhibitor of HIV integrase and envelope gp1208. Similarly, the curcuminoids of Indian spice Curcuma longa inhibit Tat-mediated gene expression, integrase, protease and virus-cell fusion of HIV-18. Coumarins: Coumarins are phenolics with fused benzene and α-pyrone rings. They can stimulate macrophages and thereby exert an indirect effect on viral infections. The oral anticoagulant warfarin prevents recurrences of cold sores caused by HSV-19; while 65
Pharmacologyonline 3: 64-72 (2006)
Newsletter
Chattopadhyay
4-propyldipyranocoumarins of Calophyllum lanigerum and C. inophyllum have pronounced anti-HIV activity8. The methyl groups at C-10 and C-11 and a hydrogen bond acceptor at C-12 of these coumarins are responsible for RTase inhibitor activity8,9, against non-nucleotide RTase inhibitor resistant viruses and showed synergism with lamivudine, nelfinavir and AZT8. The khellatone coumarin suksdorfin of Lomantium suksdorfii inhibit HIV replication, but modifications at 3´,4´-position yielded more potent 4-MeDCK and 3-hydroxymethyl-4-methyl DCK, which inhibit both primary and drug resistant HIV-1 isolates9. Table. 1. Promising Antivirals from Ethnomedicinal plants Natural product Caffeic acid Chicoric acid Chlorogenic acid Curcumin Rosmarinic acid Calanolide A Suksderfin Baicalin Hesperidin Theaflavin Torvanol A Betulinic acid Isoborneol Oleanolic acid Prostratin Putranjivain A Ursolic acid Chrysophanic acid Chrysoplenol C Hypericin Casuarinin Geraniin Tannic acid Anolignan A Cepharanthine Harmine
Skimmianine MAP30 Meliacine TAP29 Xylanase Stevian a
Source Plantago major Echinacea purpurea Plantago major Curcuma longa Plantago major Callophyllum lanigerum Lomantium suksdorfii Scutellaria baicalensis Orange, Grape Camellia sinensis Solanum torvum O.basilicum, S.claviflorum Melaleuca alternifolia S. claviflorum Homalanthus nutans Euphorbia jolkini Geum japonicum Pterocaulum sphacelatum Dianella longifolia Hypericum perforatum Terminalia arjuna Phyllanthus amarus Camellia sinensis Anogeissus acuminata Stephania cepharantha Symplocos setchuensis Zanthoxylum chalybeum Momordica charantia Melia azedarach Trichosanthes kirilowii Panax notoginseng Stevia rebaudiana
Antiviral activity (µg ml-1)
Reference 5 8 5 8 5 8,9 9 8,16 5,10 15 11 21,30 19,22 21 23 20 17,21 24 24,30 24 8,26 26 15 29 30,34 8,31 32 35 30,35 35 8,30 30
HSV-1(15.3) b, VZV*, IV* HIV-1(40-60µM) a, g HSV-2 (86.5) a, PRV* HIV-1(100µM)a,g, f, j Influenza*, HIV (10mM) HIV-1 (0.2 µM)b,e,h, k HIV (2.6µM)b,d HIV-1 (0.5)a,d, e, i HSV, PV, PIV, RSV* Rota and CoV (3-10µM)a,f HSV-1 (9.6)a HSV (2.6)b, HIV(13)a,CVB1,EV71 HSV-1, 2 (0.06)a, dDen-2, JV* HSV*d, HIV (21.8),dRota*d HIV (< 0.132 µM)b HSV-2 (6.3µM) a, d, l HSV, ADV,CV*,EV(18 µM)a,f, PV-2(0.21)b, PV-3(0.02)b,d Picorna, Rhino, PV* HSV, HIV*e HSV-2 (1.5µM)a,l HIV-1(1.8)d, e SARS CoV (3µM)f, HIV*e,m HIV-1(60.4)a,e HIV-1(0.028) a, d,m SARS,HSV,CV* HIV (0.037µM) b,d, HSV-2h,k Measles* CMV, HIV-1(0.3 nM)b,d,e,k HSV-1, JV* HIV (0.2 nM)b,d,e,k HIV (10µM)a, e HRV, HSV-1*
IC50, bEC50, cED50, Inhibit: dreplication, ereverse transcriptase, fprotease, gintegrase, h virus-induced cytopathic effects, Ivirus entry, jcellular fusion, ksyncytium formation, l attachment and penetration, minfected cell polypeptide/proviral DNA/gene expression. *IC50/EC50/ED50 not available 66
Pharmacologyonline 3: 64-72 (2006)
Newsletter
Chattopadhyay
Flavonoids: Flavonoids are hydroxylated phenolics with C6-C3 unit linked to an aromatic ring, synthesized in response to microbial infections and are inhibitors of viral RTase, integrase and protease8. Glycyrrhizin partly interfere with virus-cell binding but flavonoids with an OH- at C-3´ inhibit viral protease, RTase and CD4/gp120 interaction; while flavones lacking OH- at C-3´ inhibit CD4/gp120 interaction8. The epicatechin inhibit HIV-1 protease while (-)-epigallocatechin 3-0gallate blocks the post-adsorption entry, protease and RTase of HIV-110. The sulfated isoflavones torvanol A and glycoside torvoside of Solanum torvum had strong antiHSV-1 activity11; while hesperidin of orange and grapes inhibit replication of HSV, poliovirus (PV)-1, parainfluenzavirus (PIV)-3 and RSV5,10. The 3-methylkaempferol is a potent inhibitor of genomic RNA synthesis of poliovirus12; while oxyresveratrol inhibit HSV-1 and HIV-113. The monoflavonoid wogonin had rapid tissue distribution and prolonged plasma elimination rate showed broad antiviral spectrum14, and thus be a candidate for anti-rabies and/or anti-encephalitis drugs. Recent reports indicated that tannic acid, theaflavin-3′-gallate (IC5010µM) and theaflavin-3,3′-digallate (IC507µM) of black tea inhibit 3C-like protease of SARS coronavirus (CoV)15. Both coronavirus and rotavirus neutralize by black tea15 and hence, theaflavins can be developed as Rotavirus and Coronavirus inhibitor. The anti-inflammatory baicalin and baicalein of Scutellaria baicalensis inhibit replication, RTase and CD4 cells entry of HIV-18,16. Similarly arctiin, genistein, daidzein and chlorogenic acid inhibit influenza virus21. These bioflavonoids block viral RTase and DNA/RNA polymerase where the degree of inhibition depends on the structure and side chain. Terpenoids and Essential Oils: Essential oils are phenolics with a C3 side chain and at a lower level of oxidation. Terpenes are isoprene enriched oils and with additional oxygen they are terpenoids. Chiang et al17 reported the broad antiviral activity of apigenin, linalool and ursolic acid from sweet basil Ocimum basilicum against HSV-1, CV-B1, adenovirus (ADV)-8 and Enterovirus 71 (EV-71), of which ursolic acid had wide spectrum and thus, be a potential candidate for
anti-RNA virus drug. The entEpiafzelechin of Cassia javanica also inhibits HSV-2 penetration and replication18. The antimicrobial preservative terpinen-4-ol of Melaleuca armillaris is virucidal19; while isoborneol inhibit glycosylation of HSV-1 polypeptides19; but diterpene putranjivain inhibit HSV-2 attachment, penetration and replication20. The ursolic acid, oleanolic acid and betulinic acid derivatives inhibit protease, RTase and gp120/gp41 of HIV-1 and replication of HSV8,21. The dihydrobetulinic acid inhibit HIV-1 (EC500.9µM), while its esterification at C-3 yielded 3-O-3,3′-dimethylsuccinyl betulinic acid (DSB) that block capsid formation in wild and drug-resistant HIV-1 strains, and is under phase II clinical trial21. Similarly, oleanolic acid inhibit herpes and retrovirus replication, but its esterification at C-3 resulted in improved HIV protease activity (EC50 0.0039 µg/ml)21. The triterpenoid oleanane saponins inhibit viral DNA synthesis, while the ursane saponins inhibit capsid protein synthesis21. 67
Pharmacologyonline 3: 64-72 (2006)
Newsletter
Chattopadhyay
The sandalwood oil had a dose dependent anti-HSV-1 activity5, but Santolina insularis oil inhibits cell-to-cell transmission of herpes viruses22; while pulegone inhibit HSV-1 and pseudorabies virus replication5. The essential oil of Lippia junelliana and L. turbinata is virucidal against Junin virus,22, while oils of Artemisia douglasiana and Eupatorium patens inhibit HSV-1 and Dengue-25,22, indicating their usefulness in recurrent herpes infection. Actein, a triterpenoid saponin of Cimicifuga racemosa had potent anti-HIV activity23, but phorbol ester prostratin of Samoan ethnomedicine Homalanthus nutans inhibits protein kinase C and phorbol diester 12O-tetradeca -noylphorbol-13-acetate inhibits HIV-116. Intersetingly andrographolide can arrest G2 phase of HIV-infected cells where viral replication is optimal, and inhibit cell-to-cell transmission and HIV replication8. Quinones: Quinones are highly reactive aromatic coloured
compounds with two ketone substitutions. The switch between diphenol (hydroquinone) and diketone (quinone) occurs easily through oxido-reduction reactions. Quinones irreversibly bind with nucleophilic amino acids leading to the loss of protein functions, inhibit host-virus attachment and some viral enzymes24. The dianthroquinone hypericin inhibit retrovirus RTase, while chrysosplenol C inhibit picornavirus and rhinoviruses; but chrysophanol C and chrysophanic acid inhibits poliovirus replication16,24. The introduction of an aryl group at the piperazine moiety of fluoroquinolone increases its activity; while substitution of fluorine with an amine group yielded aryl-piperazinyl-6-aminoquinolones that inhibit HIV-1 replication24. This relationship may be useful for rational drug design with optimized antiviral activity. Tannins: Tannins are polymeric phenolics capable of tanning leather or precipitating gelatin from solution (astringency). These are based on either gallic acid (hydrolysable), or derived from flavonoid monomers (condensed). The hydrolysable tannins chebulagic and dehydrochebulic acid, punicalin and punicalagin of Terminalia chebula had anti-HIV activity8. The tannin-containing beverages, like green teas and red wines, can prevent a variety of illnesss as tannins can stimulate phagocytic cells, inhibit microbial proteins, viral adsorption and RTase5,8. Eugeniin and eugenol of Geum japonicum and Syzygium aromaticum block viral DNA polymerase and inhibit acyclovir-resistant HSV-1, HSV-2 and Epstein-Barr virus5. The Nepalese ethnomedicine Bergenia ligulata can block RNA and protein synthesis of Influenza virus25; while geraniin of Phyllanthus amarus inhibit HIV-1 RTase26. Casuarinin of Terminalia arjuna prevents attachment and penetration of HSV-226, but camelliatannin inhibit HIV-1 protease8; while tannin from Prunella vulgaris inhibit HIV-1 entry to CD4 cells by blocking gp4127. Several tannins suppressed HIV promoter activity, while phyllamyricin, retrojusticidin and repandusinic acid of Phyllanthus Sp. inhibit HIVRTase8.
68
Pharmacologyonline 3: 64-72 (2006)
Newsletter
Chattopadhyay
Lignans: Lignans are cinnamic acid derivatives. The dehydro-guanoferate of Kadsura matsudai had strong activity against HIV, influenza and herpes viruses28; while lignans of Rhus javanica had anti-HSV-2 activity similar to acyclovir5,16. The 3′-Omethylnordihydro-guaiafetic acid of Larrea tridentata suppresses HIV-1 replication8; but anolignans from Anogeissus acuminata showed synergistic inhibition of RTase of wild and drug-resistant HIV-1 strains29. The lignanolide of Ipomoea cairica, and arctigenin of Arctium lappa inhibit HIV proviral DNA while globoidnan A of Eucalyptus globoidea buds inhibit HIV integrase30. Alkaloids: Alkaloids are the heterocyclic nitrogen compounds that possess broad spectrum antiviral activities. The naphthylisoquinoline solamargine and michellamine isolated from Solanum khasianum and Ancistrocladus korupensis inhibit RTase and fusion of HIV, while berberine inhibits intestinal infections associated with AIDS8,30. The n-butylharmine inhibits HIV replication8 while harman of Ophirrhoza nicobarica inhibit plaque formation and eclipse phase of HSV replication31; measles virus replication was inhibited by skimmianine32. The isoquinoline thalimonine block virusspecific protein synthesis, haemagglutinin and neuraminidase influenza A33, while lycorine and homolycorine had high antiretroviral activities34. Interestingly the morphine derivatives aromoline and FK-3000 of Stephania cepharantha inhibit HIV-1 while the biscoclaurine cepharanthine inhibit HIV, SARS CoV, HSV-1, CV-B3 along with in vivo antiinflammatory, antiallergic and immunomodulating activity8,30. The broad antiviral activity of cepharanthine against both RNA and DNA viruses make it a candidate for developing new antivirals. The indolizidines, castanospermine and piperidines (1-deoxynojirimycin) inhibit HIV-glucosidase-1 activity and envelope glycoprotein-mediated fusion30. Lectins, Polypeptides and Sugar-containing compounds: The antimicrobial peptides are positively charged with disulfide bonds. Meliacine of Melia azedarach inhibit Junin virus replication, foot and mouth disease virus fusion, DNA synthesis and nucleocapsids assembly of HSV-130. Xylanase, a 15 kDa protein inhibit HIV-1 RTase, while Momordica charantia protein MRK29 inhibit RTase and p24 expression of HIV8,30. The N-acetylglucosamine- and
mannose-specific smaller lectins inhibit HIV fusion by blocking glycosylation at gp120/gp4130; while larger lectin MAP30 of Momordica charantia have RNA-glycosidase and DNA glycosylase/apurinic lyase activity, with integrase and DNA gyrase inhibition; but GAP31 (31KDa protein of Gelonium multiflorum) inhibits HIV integrase and cytomegalovirus proliferation8. The ribosome inactivating proteins (RIPs) inactivate ribosome by deadenylation of rRNA30; while trichosanthin and TAP29 of Trichosanthes kirilowii inhibit ribosome and HIV integrase35. The antiviral polysaccharides of Rhizophora apiculata (RAP) and R. mucronata (RMP) prevent HIV budding by blocking p24 expression; while Aloe polymannose inhibit capsid protein of picorna, entero and polioviruses30. 69
Pharmacologyonline 3: 64-72 (2006)
Newsletter
Chattopadhyay
The sulfated polysaccharide galactofucan inhibit HSV-2 binding and its entry into the host cell36, while the anionic polysaccharide stevian block attachment and replication of rotavirus and HSV-1, but the acidic polysaccharides inhibit HSV-2 and VSV replication30, indicating that the antiviral activity of these polysaccharides depends on molecular weight and sulfate content. Conclusions Many viral diseases are still fatal or are not curable, although some can be controlled with expensive life-prolonging drugs. Hence, safe, effective and inexpensive antivirals are the top global priorities of drug development. Furthermore, the long-term combination therapies of herpes and retroviruses may yield drug-resistant mutatants. Therefore, scientists are investigating antiviral usefulness of ethnomedicinal plants. A number of purified molecules have been identified as lead compounds due to their specific activity, low toxicity and significant structure activity relationship. Many of them interfere with RTase, protease, integrase, cellular fusion and target cell binding with complementary mechanism of action to the existing antiviral drugs. Promising results have been shown by calanolide A, 3-hydroxymethyl-4-methyl DCK and
DSB which are in phase II clinical trial with antiretroviral agents for long-term therapy. These candidates have the potential to come up as new antiviral drugs, as they can block specific cellular enzymes and virus entry into host cells, thereby helpful to counter viral drug resistance. References
1. Newman DJ, Cragg GM, Snader KM. The influence of natural product upon drug discovery. Nat Prod Rep, 2000: 17: 215-234. 2. Wagner EK, Hewlett M. J. Basic Virology. Blackwell Science, Malden, MA, USA, 1999. 3. Hegde VR, Pu H, Patel M. et al. Two antiviral compounds from the plant Stylogne cauliflora as inhibitors of HCV NS3 protease. Bioorg Med Chem Lett, 2003: 13: 2925-2928. 4. Li Y, Ooi LS, Wang H, But PP, Ooi VE. Antiviral activities of medicinal herbs traditionally used in Southern Mainland China. Phytother Res 2004: 18: 718-722. 5. Khan MT, Ather A, Thompson KD, Gambari R. Extracts and molecules from medicinal plants against herpes simplex viruses. Antivir Res, 2005: 67: 107-119. 6. Cheng HY, Lin TC, Yang CM, Shieh DE, Lin CC. In vitro anti-HSV-2 activity and mechanism of action of proanthocyanidin A-1 from Vaccinium vitis-idaea. J Sci Food Agricul, 2005: 85: 10-15. 7. Buckwold VE, Wilson RJ, Nalca A. et al. Antiviral activity of Hop constituents against a series of DNA and RNA viruses. Antiviral Res, 2004: 61: 57-62. 70
Pharmacologyonline 3: 64-72 (2006)
Newsletter
Chattopadhyay
8. Cos P, Maes L, Vanden Berghe D, Hermans N, Pieters L, Vlietinck A. Plant substances as anti-HIV agents selected according to their putative mechanism of action. J Nat Prod, 2004: 67: 284-293. 9. Yu D, Suzuki M, Xie L, Morris-Natschke SL, Lee KH. Recent progress in the development of coumarin derivatives as potent anti-HIV agents. Med Res Rev, 2003: 23: 322-345. 10. Yamaguchi K, Honda M, Ikigai H, Hara Y, Shimamura T. Inhibitory effects of (-)epigallocatechin gallate on the life cycle of human immunodeficiency virus type 1. Antivral Res, 2002: 53: 19-34. 11. Arthan D, Svasti J, Kittakoop P, Pittayakhachonwut D, Tanticharoen M, Thebtaranonth Y. Antiviral
isoflavonoid sulfate and steroidal glycosides from Solanum torvum. Phytochem, 2002: 59: 459-463. 12. Robin V, Irurzun A, Amoros M, Boustie J, Carrasco L. Antipoliovirus flavonoids from Psiadia dentata.Antivir Chem Chemother, 2001: 12: 283-291. 13. Likhitwitayawuid K, Sritularak B, Benchanak K, Lipipun V, Mathew J, Schinazi RF. Phenolics with antiviral activity of Millettia erythrocalyx and Artocarpus lakoocha. Nat Prod Res, 2005: 19: 177-182. 14. Tai MC, Tsang SY, Chang LY, Xue H. Therapeutic potential of wogonin: a naturally occurring flavonoid. CNS Drug Review, 2005: 11: 141-150. 15. Chen C-N, Lin Coney PC, Huang KK. et al. Inhibition of SARS-CoV 3C-like Protease Activity by Theaflavin-3,3′-digallate (TF3). Evid Based Complement Alternat Med, 2005: 2: 209-215. 16. Jassim SA, Naji MA. Novel antiviral agents: a medicinal plant perspective. J Appl Microbiol, 2003: 95: 412-427. 17. Chiang LC, Ng LT, Cheng PW, Chiang W, Lin CC. Antiviral activities of extracts and selected pure constituents of Ocimum basilicum. Clin Exp Pharmacol Physiol., 2005: 32: 811-816. 18. Cheng HY, Yang CM, Lin TC, Shieh DE, Lin CC. ent-Epiafzelechin-(4alpha–>8)epiafzelechin extracted from Cassia javanica inhibits HSV-2 replication. J Med Microbiol, 2006: 55: 201-206. 19. Farag RS, Shalaby AS, El-Baroty GA, Ibrahim NA, Ali, MA, Hassan EM. Chemical and biological evaluation of the essential oils of different Melaleuca species. Phytother Res, 2004: 18: 30-35. 20. Cheng HY, Lin TC, Yang CM, Wang KC, Lin LT, Lin CC. Putranjivain A from Euphorbia jolkini inhibits both virus entry and late stage replication of herpes simplex virus type 2 in vitro. J Antimicrob Chemother, 2004: 53: 577-583. 21. Yogeeswari P, Sriram D. Betulinic acid and its derivatives: a review on their biological properties. Curr Med Chem, 2005: 12(6): 657-666. 22. Garcia CC, Talarico L, Almeida N, Colombres S, Duschatzky C, Damonte EB. Virucidal activity of essential oils from aromatic plants of San Luis, Argentina. Phytother Res, 2003: 17: 1073-1075. 71
Pharmacologyonline 3: 64-72 (2006)
Newsletter
Chattopadhyay
23. Sakurai N, Wu JH, Sashida Y. et al. Anti-AIDS agents. Part 57: Actein, an antiHIV principle from the rhizome of Cimicifuga racemosa, and the anti-HIV activity of related saponins. Bioorg Med Chem Lett, 2004: 14: 1329-1332. 24. Richter S, Parolin C, Palumbo M, Palu G. Antiviral properties of quinone-based drugs. Curr Drug Targets Infect Disord, 2004: 4: 111-116. 25. Rajbhandari M, Wegner U, Schopke T, Lindequist U, Mentel R. Inhibitory effect of Bergenia ligulata on influenza virus A. Die Pharmaz, 2003: 58: 268-271. 26. Notka F, Meier G.R, Wagner R. Inhibition of wild-type HIV and reverse transcriptase inhibitor-resistant variants by Phyllanthus amarus. Antivir Res, 2003: 58: 175-186. 27. Liu S, Jiang S, Wu Z, Lv L, Zhang J, Zhu Z, Wu S. Identification of inhibitors of the HIV-1 gp41 six-helix bundle formation from extracts of Chinese herbs Prunella vulgaris and Rhizoma cibotte. Life Sci, 2002: 71: 1779-1791. 28. Kuo YH, Li SY, Huang RL, Wu MD, Huang HC, Lee KH. Schizarin B, C, D and E, four new lignans from Kadsura matsudai and their antihepatitis activities. J Nat Prod, 2001: 64: 487-490. 29. Chattopadhyay D, Naik TN. Antivirals of Ethnomedicinal Origin:Structure-activity Relationship and Scope. Mini Rev Med Chem, 2006 (in press). 30. Ovenden SP, Yu J, Wan SS. et al. Globoidnan A: a lignan from Eucalyptus globoidea inhibits HIV integrase. Phytochem , 2004: 65: 3255-3259. 31. Chattopadhyay D, Arunachalam G, Mandal AB, Bhattacharya SK. Dose dependent therapeutic antiinfectives from ethnomedicines of Bay Islands. Chemother, 2006: 52: 151-157. 32. Olila D, Olwa-Odyek A, Opuda-Asibo J. Screening of Zanthoxylum chalybeum and Warburgia ugandensis for activity against measles virus (Swartz and Edmonston strains) in vitro. J African Health Sci, 2002: 2: 2-10. 33. Serkedjieva J, Velcheva M. In vitro anti-influenza virus activity of the pavine alkaloid (-)-thalimonine isolated from Thalictrum simplex L. Antivir Chem Chemother, 2003: 14: 75-80. 34. Szlavik L, Gyuris A, Minarovits J, Forgo P, Molnar J, Hohmann J. Alkaloids from Leucojum vernum and antiretroviral activity of Amaryllidaceae alkaloids.Planta Med, 2004: 70: 871-873. 35. Wang JH, Nie HL, Huang H, Tam SC, Zheng YT. Independency of anti-HIV-1 activity from ribosome-inactivating activity of trichosanthin. Biochem Biophys Res Commun, 2003: 302: 89-94. 36. Thompson KD, Dragar C. Antiviral activity of Undaria pinnatifida against herpes simplex virus. Phytother Res, 2004: 18: 551-555.