Jacobsen’s Method of Epoxidation of an Alkene
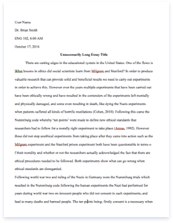
- Pages: 17
- Word count: 4064
- Category: Chemistry
A limited time offer! Get a custom sample essay written according to your requirements urgent 3h delivery guaranteed
Order NowVarious types of reactions were completed to first create and then use Jacobsen’s catalyst in the asymmetric epoxidation of an unknown alkene with bleach in the laboratory. The chiral epoxide synthesized was then characterized with GC/MS and NMR. With this information the unknown alkene was able to be identified as 4-chlorostyrene. Introduction
Organisms have evolved with mechanisms that use specific enantiomers of molecules. If the chirality of the molecules is incorrect, they may not be utilized or may even hurt the organism. For this reason a method to create chiral molecules is very important and for this reason we study asymmetric synthesis. One method in which a chiral epoxide can be synthesized is through the use of a Jacobsen-type catalyst. In order to synthesize Jacobsen’s catalyst, Jacobsen’s ligand must be created first which requires the use of 3,5-di-tert-butyl-salicylaldehyde . There are many methods by which this salicylaldehyde can be synthesized but one method with a relatively high yield starts with 2,4-di-tert-butylphenol. The reaction scheme is shown below in Figure 1.
Figure 1: (1) 2,4-di-tert-butylphenol ,(2) 2,4-di-tert-butyl-6-hydroxymethylphenol, (3) 3,5-di-tert-butyl-salicylaldehyde shown above. In this reaction (1) was reacted with formaldehyde utilizing a Lederer-Mannase reaction giving (2) with a good yield. This compound was then oxidized in order to form (3). One oxidizing agent which could be used is sodium hypochlorite (bleach) with phase transfer catalyst. This method of synthesizing the salicylaldehyde is advantageous because it has a very high yield of 88%.1 It also uses many of the techniques that undergraduate students have already learned such as vacuum filtration, drying over anhydrous sodium sulfate, and recrystallization. An alternative method in which one can synthesize chiral epoxides is through the use of a fructose-derived catalyst instead of the Jacobsen’s catalyst. The dioxirane that is formed in the derivation performs the oxidation. The reaction scheme for creating the fructose derived catalyst is shown below in Figure 2.
Figure 2: (4) D-fructose , (5) bis-ketal alcohol, (6) fructose-derived catalyst
The first step in synthesizing the catalyst is ketalization, which protects four of the five hydroxyl groups on the fructose. This ketalization allows for good stereoselectivity having an enantiomeric excess value ranging from 85-97%.2 The second and final step is the oxidation of the unprotected hydroxyl group using PCC, an oxidizing agent. The newly created catalyst can then be converted into a dioxirane, as shown in Figure 3, in the presence of potassium peroxomonosulfate. The dioxirane formed performs the epoxidation of the alkene.
Figure 3: Catalyst – top middle, dioxirane – bottom middle, alkene and epoxide – right side.
Though the yield of this reaction is high it utilizes percholoric acid and PCC which are potentially hazardous reagents. Other than that, the reaction allows the student to use such techniques as recrystallization to purify products, column chromatography to separate products from reactants, and allows students to propose designs of other catalysts that could be used employing different sugars and expected yields and enantiomeric excesses. Results/Discussion
Resolution:
In order to undergo asymmetric synthesis, the reactants must be as optically pure as possible to produce a product with high optical purity, but because a single enantiomer of 1,2-diaminocyclohexane is much more expensive and impractical for undergraduate laboratories, a mixture of trans-cis isomers is used. Therefore a resolution step is required to produce higher optical purity. The problem with using simple steps such as recrystallization is that enantiomers have the same physical properties. To circumvent this problem, diastereomeric tartrate salts are synthesized by mixing with (L)-(+)-tartaric acid. These diastereomers happen to have large enough differences in their solubilities to be able to separate them via recrystallization. This difference in solubility is likely due to stronger ionic interactions between the tartaric acid and the diammonium with the matching configuration.
When running the experiment it is important to add the diamine to the tartaric acid in neat form because the reaction is exothermic and the heat could hinder the reaction. Glacial acetic acid is then added to lower the pH of the solution to protonate the diamine allowing it to precipitate and form crystals to be isolated. The literature yield in the reaction is 30% of the (R,R)-diammonium salt with pure diamine with a melting point range from 205-207°C and an optical rotation of -12.5°.3 The resolution reaction scheme is shown below in Figure 4.
Figure 4: 1,2-Diaminocyclohexane with the addition of (L)-(+)-tartaric acid reacts to create a four diastereomers but only the (1R, 2R)-diammonium-(2R, 3R)-tartrate salt form crystals in cold water.
In the experiment a yield of 28% of the target salt was resolved. This yield is very good when compared to the theoretical maximum of 30%. The small loss of product may be due to pipet error or residue left in Hirsch funnel. It also may be because the entire product had not precipitated out before being vacuum filtrated. The observed melting point had a broader range of 200-206°C which may have been due to impurities in the sample. Finally an optical rotation of -12.6 was found with a 1% concentration of the salt in distilled water. This optical rotation is very close to the literature value showing that the resolution had very high enantiomer excess and had been successful. Jacobsen’s Ligand Synthesis
The synthesis of Jacobsen’s ligand is a preliminary step towards creating the catalyst needed for epoxidation. The tartrate salt is dissolved in a mixture of potassium carbonate and water in order to deprotonate the ammonium function. Because there are two ammonium functions, two equivalents of the potassium carbonate are needed. Adding ethanol lowers the polarity of the solution which, in turn, increases the solubility of the salicylaldehyde allowing it to stay in solution and react with the diamine to form the ligand. Solubility of the ligand can be decreased by adding water to allow the ligand to precipitate and form crystals when cooled to be isolated via vacuum filtration. Crude ligand product is dissolved in a solvent mixture of ethyl acetate and hexane because it does not dissolve reasonably in either solvent alone. The mixture of solvents allows for a better solubility curve which will increase the purity of the final ligand product. This process yields nearly 100% of the target product.4 Jacobsen’s ligand has a melting point of 202-203°C.5 It also has an optical rotation of -315 ± 15 in methylene chloride.6 The reaction scheme is shown below in Figure 5.
Figure 5: tartrate salt (left) is reacted with 2 equivalents of potassium carbonate to release the diamine (center) which can then attack the carbonyl of the aldehyde to create Jacobsen’s ligand (right).
The experimental yield of the ligand was 65% a rather low yield when compared to the literature value of nearly 100%, which may have been due to loss when trying to extract the product from the round bottom flask and the Hirsch funnel after vacuum filtration. Also there may have been some unreacted aldehyde which did not stay in solution to react with the tartrate salt attributing to the low yield. Another reason for the low yield may have been from all the product not crystallizing prior to vacuum filtration. The observed melting point was 200-205°C which is very close to the literature value of 202-203°C, which provides some evidence that the product obtained was pure. The broader range may have been due to several impurities left in the product such as unreacted tartrate salt or salicylaldehyde. However the optical rotation of the product was -328° which is within the error of the literature value. Jacobsen’s Catalyst Synthesis
After the ligand was synthesized, the catalyst for epoxidation could finally be made. Manganese(II) acetate is crushed in order to increase the surface area of the particles allowing for more oxidation to occur faster by maximizing the number of collisions. The manganese(II) ion forms a chelate with the two oxygen atoms of the ligand and coordinates the imine nitrogen atoms as well to form a manganese(II) complex. After this complex is formed oxygen is introduced into the system via an air stream through a glass tube in order to oxidize the manganese(II) to manganese(III) in presence of lithium chloride and the dark-brown Jacobsen’s catalyst is formed. The reaction progress is monitored by TLC. The polarity of the solution is lowered by adding high-boiling petroleum ether and evaporating excess solvent allowing the catalyst to precipitate and be collected. Figure 6: Jacobsen’s ligand (left) reacted with manganese(II) acetate in water to form a coordination complex (middle) which is oxidized via an air stream and addition of lithium chloride (right).
In the laboratory a 41% yield was observed which was rather far from the literature value of 70-85%.7 Loss of product could be due to allowing the catalyst to sit in the high boiling petroleum ether over the weekend because of lack of time to finish it in one laboratory period. Also the ligand was not completely used up as some yellow solid could still be seen in the catalyst solution after stopping the reflux. One problem experienced in the lab was the solvent being boiled off too quickly during the reflux. After adding ethanol, within five minutes the solvent level would drop significantly. In order to alleviate this problem the heat was turned down to create a gentler boil and more ethanol was added. In the TLC a very faint spot near the solvent front was still seen after stopping the reaction signifying that unreacted ligand was still present. A dark spot near the start line signified the presence of catalyst. The melting point range of the product obtained was 327-330°C which is close to the literature value of 330-332°C. Epoxidation of an Alkene
Jacobsen’s catalyst was used to catalyze the epoxidation of an unknown alkene with a bleach solution that was buffered in order to maintain a pH of 9.5-11.5. This pH minimizes the amount of side reactions that could occur such as hydrolysis of the epoxide. After the reaction is completed it is extracted and purified with flash chromatography to separate the epoxide from unreacted alkene and other side products.
Figure 7: 4-chlorostyrene (left) is reacted with sodium hypochlorite in the presence of Jacobsen’s catalyst to create 3-chlorostyrene oxide (right) In the lab a yield of 18.9% epoxide was accomplished. Which is quite close to the literature value of 22%.8 A higher yield could have been given if the temperature was higher however the mixture would then be more susceptible to side reactions which would most likely decrease the purity of the product. The enantiomeric excess calculated using the GC of the epoxide sample was found to be 41.4%. The TLC used to monitor the reaction was a 1:7 mixture of ethyl acetate:hexane, giving an Rf value of around .5 for the epoxide and .8 for the alkene. Both of these compounds were visible under UV-light. Another band with an Rf of around .3 was also visualized under UV-light which may have been due to some side product such as a diol formed by the degradation of the epoxide. Experimental
Resolution
In the resolution step of the experiment 2.24 g (0.01 mol) of (L)-(+)-tartaric acid was dissolved in 15 mL of distilled water with a stir bar in a 150 mL beaker. 6 mL (0.05 mol) of a 60:40 (trans:cis) mixture of 1,2-Diaminocyclohexane was then slowly added while stirring the system. After 10 minutes, 5 mL glacial acetic acid was added. After a few more minutes the solution was placed in an ice bath for 30 minutes. Solid was collected by vacuum filtration and washed with 5 mL ice-cold distilled water twice and 5 mL ice-cold methanol twice. The white product was dissolved again with 100 mL hot water and allowed to reach room temperature before placing in an ice-bath. This experiment produced 3.77 g of tartrate salt or a 28% yield. The observed melting point obtained was 200-206°C and the optical rotation was found to be -12.6° with a concentration of 1.008%. The tartrate salt appeared to be fine, white, shiny, odorless crystals. Ligand Synthesis
In the ligand synthesis step of the experiment 0.32 g (0.001 mol) tartrate salt, 4 mL (0.22 mol) water, and .33 g (0.002 mol) K2CO3 were mixed in a 100 mL round bottom flask. 4 mL of 95% ethanol was added and the solution was refluxed. While stirring, 0.56 g (0.002 mol) 3,5-di-tert-butyl-salicylaldehyde was mixed with 3 mL of 95% ethanol in a warm water bath to help the aldehyde dissolve. This solution was then added to the round bottom flask and rinsed with 95% ethanol via a Pasteur pipette. After refluxing for 45 minutes, 3 mL water was added and then the solution was taken off the heat and allowed to cool to room temperature. After reaching room temperature, the solution was placed in an ice bath and after 10 minutes, the crystals were collected via vacuum filtration washing with 3 mL ice-cold ethanol two times. The solid was extracted and dissolved with 15 mL of a mixture of ethyl acetate:hexane (1:1) and washed with 15 mL water twice and 30 mL saturated sodium chloride. The yellow solution was then dried over anhydrous sodium sulfate for 10 minutes and then the solvent was removed using the rotary evaporator. This experiment produced 0.4211 g Jacobsen’s ligand or a yield of 65%, a melting point of 200-205°C, and an optical rotation of -328°. The ligand appeared to be a bright yellow solid. Catalyst Synthesis
In the catalyst synthesis step 0.40 g (0.0007 mol) of ligand was dissolved in 15 mL ethanol and refluxed for 10 minutes in a two-necked round bottom flask with a water-jacketed condenser attached. 0.30 g (0.001 mol) of crushed manganese(II) acetate was added and the solution was refluxed for an additional 20 minutes. Air was then introduced into the system via a glass tube through an adapter. 15 mL ethanol was added as the solution level became too low. As the yellow ligand continued to disappear the solution became a darker shade of brown. The reaction was monitored by TLC using a prepared diluted solution of the ligand in ethyl acetate:hexane (1:4) mixture and a small amount of diluted reaction mixture. Once the ligand spot disappeared from the reaction mixture, 0.10 g (0.002 mol) lithium chloride was added and the mixture was refluxed for 15 more minutes.
Then the flask was removed from the heat and the excess solvent was removed with the rotary evaporator. The residue was dissolved in ethyl acetate and washed with 10 mL water twice and 20 mL saturated sodium chloride. This mixture was dried over anhydrous sodium sulfate for 10 minutes before being extracting from the drying agent and adding 20 mL high-boiling petroleum ether. Solvent removed again with rotary evaporator leaving a brown slurry. Because of time constraints this slurry was left for a few days before being vacuum filtrated and obtaining the solid precipitate. This experiment produced 0.19 g of Jacobsen’s catalyst or a 41% yield with a melting point of 327-330°C. The catalyst appeared to be a dark-brown solid. Epoxidation of an Alkene
In the epoxidation step a buffered bleach solution was created by mixing 12.5 mL (0.19 mol) sodium hypochlorite, one drop of 1M NaOH, and 10 mL of 0.05M Na2HPO4. Another solution with 0.5188g (0.004 mol) 4-chlorostyrene, 0.1882g of Jacobsen’s catalyst, and 5 mL ethyl acetate was then created and added to the buffered bleach solution. The flask was sealed with Parafilm and stirred vigorously. After about an hour the reaction was monitored by TLC using a 1:7 mixture of ethyl acetate:hexane to determine when the 4-chlorostyrene was completely used up. Once the reactant was completely consumed, 50 mL of hexane was added to work-up the mixture. The two phases were then separated and the organic layer was extracted twice with 10 mL of saturated NaCl. The organic extract was then dried over anhydrous sodium sulfate. Once dry, it was transferred to a round bottom flask and rotary evaporated to remove the solvent. The yellow oily residue was suspended in 10 mL of hexane and filtrated using a Pasteur pipette with a small amount of cotton on the bottom. Finally the solution was rotary evaporated once more until viscous oil with a glue-like odor remained. This would later be used for flash chromatography to further purify and isolate the epoxide from the unreacted alkene and side products. Identification| Chemical shift (ppm)|
Phenolic-H| 13.6|
N=C-H| 8.4|
N-C-H| 3.4|
Cyclohexane| 1.6|
Tert-butyl| 0.8|
After the chromatography column was made it was wet with hexane and pretreated with 20 mL 1% triethylamine in hexane. The column was then rinsed with 20 mL of hexane and the crude was then added dissolved in a small amount of hexane. A 1:7 solvent mixture of ethyl acetate:hexane was used to elute ten fractions with 10 mL each. TLC was used to identify which fractions contained the epoxide. These fractions were then combined and rotary evaporated to remove the solvent. This experiment produced 0.1052 g of epoxide or a 18.9% yield with an enantiomeric excess of 41.4%. The epoxide appeared to be a viscous, yellow oil. Spectra Discussion
Ligand – H-NMR
Intramolecular hydrogen bonding causes the phenolic-H to be shifted down to 13.6 ppm from normal phenolic protein shifts of 4-8 ppm.10 Also because of the proximity of the nitrogen, the imine-H is shifted down to lower field as well to 8.4 ppm.
Ligand – C-NMR
Because the solution used for C-NMR is highly diluted, noise prevents the pertinent peaks from being easily visualized. The electronegativity of the nitrogen in the imine and its hybridization cause the C=N imine carbon to have a chemical shift of 166 ppm. Ligand – Infrared
Identification| Wavenumber (cm-1)|
C=N| 1630|
O-H| 2400-3000|
sp3 C-H| 2950|
The pertinent peaks in the obtained IR are the C=N bond at 1630 cm-1, a broad O-H peak from 2400-3000 cm-1 which is shifted to lower wavenumbers due to intramolecular hydrogen bonding between the phenolic OH hydrogen and the imine nitrogen, and a strong peak at 2950 cm-1 for the sp3 C-H group. There appears to be two additional peaks at 1361 and 1438 cm-1 which may be due to C-H bending and puckering by the benzene ring shifted from the normal 1600 and 1500 cm-1 due to the weakening of bonds by the electronegative hydroxyl group.11 There are also two peaks at 2180 and 2017 cm-1 which may be due to presence of unreacted aldehyde. Ligand – UV-Vis
UV-Vis was done with a concentration of 10-4 M and absorbencies can be seen at wavelengths of 270 nm and 330 nm. There was a strong absorbance detected at around 270 nm due to the π-π*-transitions of the benzene rings while the medium absorbance detected at 330 nm can be attributed to the π-π and n-π*-transitions of the C=N. Catalyst – Infrared
Identification| Wavenumber (cm-1)|
C=N| 1604|
sp3 C-H| 2950|
The C=N peak in the catalyst IR is shifted down even further due to the weakening of the bond due to the coordination of the nitrogen of the imine with the manganese ion. Also in this spectrum the broad O-H stretch is now absent which supports the reaction being complete and the ligand being depleted. The sp3 C-H stretch is still visible since it is still present in the catalyst. Also the peaks at 2180 and 2017 cm-1 in the ligand IR seemed to have disappeared through the process showing that further purification was done in the process of synthesizing the catalyst. Catalyst UV-Vis
The UV-Vis for the catalyst was done with a concentration of 2.56 * 10-5 M. Three peaks of strong absorbencies can be seen at 200, 224, and 242 nm. These peaks are most likely due to π-π* transitions in the phenyl rings of the catalyst and the imine function. The lack of distinct peaks at around 496 nm and 436 nm show that the sample may have been too diluted as these peaks are the ones which give the catalyst its distinctive dark brown color due to ligand field and LCMT absorptions. Epoxide H-NMR
The H-NMR spectrum of the epoxide shows two doublets with an integration of 4 at a chemical shift of around 7.2 ppm indicating the presence of a para-substituted arene. At a chemical shift of 2.5-4 ppm there are three doublets with an integration of 1 each indicative of a mono-substituted epoxide. Between 0.5 to 2 ppm there appears to be a lot of noise which may be due to impurities in the sample. These results are very similar to the literature spectra with three doublets at 2.68, 2.6, and 3.07 ppm for the epoxide hydrogens and a multiplet at 7.12-7.26 ppm for the arene.12
Epoxide C-NMR
The C-NMR spectrum shows four signals (2 large and 2 small) around 130 ppm which further supports the idea of a para-substituted arene. There are also two signals at around 51 ppm which are due to an asymmetric epoxide. Finally there are some small peaks from 14-30 ppm which are probably again due to impurities. Again these are very similar to the literature which has peaks at 51.4, 51,9 and 4 peaks around 130 ppm.12 Epoxide GC
The GC of the epoxide gave four peaks at retention times 21, 26, 28, and 32 respectively. The peak with the shortest retention time is most likely due to unreacted alkene. The peak at 32 or the longest retention time is most likely due to an aldehyde or a ketone made via rearrangement of the epoxide. The peak at 26 has a height of 1300000, with a width of 1.3 while the peak at 28 has a height of 700000, with a width of 1. Using these values the enantiomeric excess was found to be 41.4%. Epoxide MS
The mass spectrums of the epoxide appear to have some impurities as peaks appear above the molecular weight of 154 g/mol which appears in the literature.13 These peaks may be due to some type of side reactions such as the formation of a dimer or may include degraded parts of the catalyst which were not separated from the epoxide completely. From the mass spectrum it is evident that a chlorine is present as a peak at about one-third height appears two mass per charge units higher than most of the larger peaks. The base peak appears to be 89.1 m/z for the epoxide with a molecular ion peak at 154.0 m/z. Conclusion
In this experiment asymmetric epoxidation of an unknown alkene was performed. After characterization of the final product I was able to identify which alkene was initially used. This project taught me the basics of epoxidation and how to read spectra to identify a molecule. It also taught me many of the techniques used in laboratories and prepared me for a future career in research. It has taught me how to research different topics utilizing different types of resources. This paper itself has given me experience in writing a formal report and has familiarized me with the format in which it should be written to be seen as both professional and educational.
References
1. Cepanec, Ivica, Mikuldas, Hyvoje and Vinkovic, Vladimir, An Improved Method for Synthesis of Jacobsen’s Catalyst, Synthetic Communications, 31: 19, p. 2913-2919. 2. Burke, Andy, Dillon, Patrick, Martin, Kyle and Hanks, T. W., Catalytic Asymmetric Epoxidation Using a Fructose-Derived Catalyst, J. Chem. Educ., 2000, 77 (2), p. 271. 3. Larrow, Jay F., Jacobsen, Eric N., Gao, Yun, Hong, Yaping, Nie, Xiaoyi, and Zepp, Charles M., Journal of Organic Chemistry, 1994, vol. 59, 7, p. 1939-1942 4. Nakano, Tamaki, Yade, Tohru, and Okamoto, Yoshio. Macromolecules, 2003, vol. 36, 10, p. 3498-3504. 5. Yao, xiaoquan, Qiu, Min, Lue, Weiran, Chen, Huilin, Zheng, Zhuo, Tetrahedron: Asymmetry, 2001, vol. 12, 2, p. 197-204. 6. Deng, L. Jacobsen, E.N. J. Org. Chem., 1992, 57, p. 4320. 7. Robert, Anne, Tsapara, Anna, Meunier, Bernard, Journal of Molecular Catalysis, 1993, vol. 85, p. 13-20. 8. Turk, Hayrettin, Ford, Warren T., Journal of Organic Chemistry, 1991, vol. 56, 3, p. 1253-1260. 9. Hanson, J., J. Chem. Educ. 2001, vol. 78, 9, p. 1266
10. Bacher, Alfred D., Organic Chemistry 30CL Reader, 2011, p. 44 11. Reusch, William H. “Infrared Spectroscopy.” Michigan State University Department of Chemistry. Web. 2011. <http://www2.chemistry.msu.edu/faculty/reusch/VirtTxtJml/Spectrpy/InfraRed/infrared.htm>. 12. Magerlein, Woflgang, et al. Patent: US2006161011A1, 2006. 13. Gelalcha, Feyissa, et al. Chemistry—A European Journal, 2008, vol. 14, 25, p. 7687-7698.