Zeta Potential of Shape-controlled TiO2 Nanoparticles with Surfactants
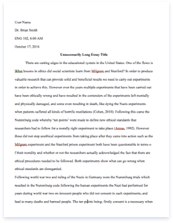
- Pages: 11
- Word count: 2598
- Category: Control
A limited time offer! Get a custom sample essay written according to your requirements urgent 3h delivery guaranteed
Order NowAbstract:
To assess the stability of TiO2 nanoparticles in aqueous suspensions, zeta potential of shape-controlled TiO2 nanoparticles with surfactants was measured, leading to a unique finding; that the TiO2 nanoparticles shaped by SDS have two isoelectric points, while other shape-controlled TiO2 nanoparticles have only one isoelectric point. Shape-controlled TiO2 nanoparticles have a more negative zeta potential than TiO2 nanoparticles obtained without the addition of surfactants during synthesis and commercial anatase TiO2 nanoparticles P-25. The results suggest that the zeta potential of TiO2 nanoparticles can be manipulated during the synthesis processes.
Key words: Zeta potential, TiO2 nanoparticles, Shape controlling, Surfactant
- Introduction
The stability of dispersions of inorganic particles in aqueous phase plays an important role in industrial and science research. Surface charge and stability of TiO2 nanoparticles in water have been studied in a variety of systems, however, the role of specifically adsorbed ions on the nanoparticles is not clearly understood [1, 2]. With the particular interests in photocatalytic reactions, TiO2 nanoparticles have become one of the most important materials to use for the removal of organic contaminants from water. In addition to which, extensive work has been carried out showing that many organic contaminants can be mineralized into carbon dioxide and water on UV irradiated TiO2 nanoparticle suspension [3].
There are many complicated steps involved in the photocatalytic reaction processes, but the key is the preparation of suspensions with a high degree of homogeneousness and stability. The viability of the organic contaminant removals can be enhanced by increasing the stability of nanoparticles in the suspension and the adsorption of various organic molecules onto the TiO2 nanoparticle surfaces [4].
In an aqueous system, nanoparticles always carry charges because of the ionization, the adsorption of ions or the preferential substitution of ions from the particles surface [5, 6]. The physical properties of TiO2 nanoparticles suspensions are mostly dependent on the behavior of aqueous suspensions, which are especially reactive to the electrical and ionic structure of the particle-liquid interface.
Zeta potential of nanoparticles is often used to evaluate the variety and intensity of the charge on the particles in suspensions. This is a very important index which reflects the intensity of repulsive force among particles and the stability of dispersion. Zeta potential is crucial on the stability control of TiO2 nanoparticles in suspensions and the adsorption properties of TiO2 nanoparticle surface [5, 6].
Studies have been done on the stability and zeta potential of titanium dioxide nanoparticle suspensions [7, 8]. Mandzy et al. [8] studied the effects of ultrasonic on the agglomeration of TiO2 nanoparticles suspension. They found that ultrasonic breakage could prevent the reagglomeration by controlling zeta potential. Gumy et al. [9] demonstrated that the isoelectric points could be correlated to with the photocatalytic activity of TiO2 particles and that the surface charge of TiO2 particles affected the inactivation kinetics of bacteria significantly. Palarick et al. [10] studied the influence of pH on the zeta potential of titanium dioxide particles in different dispersion environments.
They observed that the zeta potential and value of the isoelectric point of each sample and that different chemical composition had different dependence on pH values. Lebrette et al. [11] performed an extensive study to evaluate the influence of ethanol on the stability of aqueous TiO2 suspensions. They found alcohol addition strongly affected zeta potential values of particles and increases in suspension viscosity without influence on the surface charge. The realistic interpretation between alcohol molecules could reduce the repulsive potential between particles that leads to suspension destabilization.
In this research, zeta potential of shape-controlled TiO2 nanoparticles synthesized from Ti(OBu)4 and TiCl4 was studied comprehensively. Smoluchowski equation was chosen to calculate the zeta potential of TiO2 nanoparticles under the action of an electric field. The effect of SDS on the zeta potential of TiO2 nanoparticles is also investigated and as a result of which it is concluded that the zeta potential can be manipulated through shape control process.
- Experiment
Shape controlled TiO2 nanoparticles were prepared with a sol-gel method with surfactants. Titanium butoxide was dissolved in ethanol with a titanium/ethanol molar ratio 1:10, the pH of the solution was then adjusted to 2 with HCl. When TiCl4 was used as titanium source, deionized water was used as solvent with a molar ratio titanium:water=1:10. Surfactants were dissolved in ethanol and fed into the titanium precursor solution slowly with a molar ratio titanium:surfactant=1:1, and a feeding speed of 0.5ml/min. Next, a titanium/surfactant mixture was fed into a blend of deionized water/ethanol (titanium:water:ethanol=1:4:10 molar ratio, 0.5ml/min). Hydrolysis reaction and polymerization took place in this mixture with the result that TiO2 sol was formed. After gelation for 24 hours, the gel was dried at 700C in an oven until yellow crystal was obtained.
This was then treated in a muffle furnace at high temperatures, after which white TiO2 nanoparticles were obtained. The Nanoparticles were then dispersed in double distilled and deionized water and the suspension was treated in an ultrasonic bath for one hour. The pH of this suspension was adjusted to set points with HCl or NaOH. Zeta potential of the samples was studied with Zetacompact Z8000 model (CAD Instrumentation). Smoluchowski equation was chosen to calculate the zeta potential of TiO2 nanoparticles. The electric field added on the suspension was controlled by the cell voltage of Zetacompact Z8000 model and was fixed at 80v.
- Results and discussions
3.1 Zeta potential measurement of TiO2 nanoparticles prepared from Ti(OBu)4
Surface charge of TiO2 nanoparticles in aqueous suspensions can be determined by factors such as pH. The zeta potential of TiO2 nanoparticle in suspensions is probably controlled by the pH through the following process [4]:
Figure 1 shows the zeta potential vs. pH curves of shape-controlled TiO2 nanoparticles, no shape-controlled TiO2 nanoparticles and P-25. All the nanoparticles in Figure 1 were prepared from Ti(OBu)4 and calcited at 5000C.
The isoelectric point (IEP) of non shape-controlled TiO2 nanoparticles and P-25 in deionized water is about 6.5 and 7 respectively, as shown in Figure 1, which is in agreement with the results from other researchers [13]. Significant lower isoelectric points can be observed for shape-controlled nanoparticles. IEP of shape controlled TiO2 nanoparticles is shifted by 3 and 4 pH units to 4.2 and 3 when nanoparticles were shape controlled by DBS and cellulose respectively.
For each shape-controlled nanoparticle, there is a specified zeta potential curve and IEP. The overall zeta potential of shape controlled nanoparticles decreases as the pH increased. The observed lower IEP of the shape controlled nanoparticles result from the lower surface charge and the increase of negatively charged –TiO– groups.
The change may be also due to the blockage of active sites on the nanoparticle surface and the difference in the IEP and curve is probably due to the surface area and the number of adsorptive sites on the surface of the nanoparticles. The shape control process can alter the surface energy of the nanoparticles, which results in different adsorption and affinity of the protons on the surface.
Figure 1, Zeta Potential of the shape controlled TiO2 nanoparticles prepared from Ti(OBu)4
Zeta potential vs. pH curves of TiO2 nanoparticles shaped by SDS and treated with different temperatures (5000C-8000C) are illustrated in figure 3. An odd phenomenon was seen in Figure 2 with the use of SDS. The pH-Zeta potential curve has a second IEP and reenters the positive zeta potential zone; this does not happen with TiO2 synthesized with TiCl4 and SDS as seen in Figure 3. This phenomenon was not limited to a specific sample or preparation condition.
A similar trend of pH-Zeta potential curves can also be seen for the TiO2 synthesized at 500 °C, 600 °C, 700 °C, and 800 °C therefore excluding any possibility that it was a bad grouping of data, as shown in Figure 2. This phenomenon can not be explained without knowing what functional groups are attached to the titanium skeleton or surface, however what can be said, is that the overall zeta potential shifts to the right as the pH increases but that it is still lower then the IEP of P-25.
The first IEP of the TiO2 nanoparticles shape-controlled by SDS appears at pH between 2.7 to 3.3 with treatment temperature increased from 5000C-8000C. Zeta potential becomes negative when pH surpasses the first IEP and then reenters the positive charged zone when pH surpasses 4. The minimal zeta potential values can be found at pH 3.5.
Figure 2, Zeta potential of SDS shape controlled TiO2 nanoparticles prepared from Ti(OBu)4
Figure 3 FTIR spectra of shape-controlled TiO2 nanoparticles before and after calcination at 400oC.
Figure 3 shows the FTIR spectra of the TiO2 nanoparticles synthesized with SDS. Spectra 1 and 2 represent the TiO2 nanoparticles which were treated at 4000C and 700C respectively. Figure 3 indicates that there were no organic functional groups or material residuals attaching on the nanoparticle surface after the nanoparticles were treated at 4000C. The result excludes the possibility that the second IEP was caused by the functional groups attached on the surface.
3.2 Zeta potential measurement of TiO2 nanoparticles prepared from TiCl4
Figure 4 shows zeta potential vs. pH curves of shape-controlled TiO2 nanoparticles prepared from TiCl4. IEP of shape-controlled nanoparticles in Figure 4 was higher than the IEP of the TiO2 nanoparticles synthesized from Ti(OBu)4 shown in Figure 1. The same results also found that the shape-controlled TiO2 nanoparticles had lower IEP than the no shape-controlled TiO2 nanoparticles and P-25. Figure 5 shows the pH-Zeta potential curves of TiO2 nanoparticles shape controlled by SDS prepared from TiCl4.
The nanoparticles were treated at 5000C to 8000C. IEP of the nanoparticles increased from 4.8 to 8 when the treatment temperature increased from 5000C to 8000C. The comparison of the zeta potential prepared from Ti(OBu)4 and TiCl4 with using SDS is shown in Figure 6. Nanoparticles prepared from Ti(OBu)4 have two IEP at pH 3 and 3.8, while the nanoparticles prepared from TiCl4 have only one relative higher IEP at pH 4.7.
Figure 4, Zeta Potential of the shape controlled TiO2 nanoparticles prepared from TiCl4
Figure 5, Zeta potential of SDS shape controlled TiO2 nanoparticles prepared from TiCl4
Figure 6, Comparison of SDS shape controlled TiO2 nanoparticles prepared from Ti(OBu)4 and TiCl4
3.3 Zeta potential measurement of TiO2 nanoparticles in SDS solution
In order to study how to improve the stability and homogeneity of TiO2 nanoparticle suspension, zeta potential of TiO2 nanoparticles in SDS solution was studied by using a 0.5mM solution of SDS. The effect of a shape-controlled process on zeta potential in the deionized distilled water have all shifted the IEP to the left and mostly have a shorter period where the colloid is positive; the descent to negative zeta potential is steep and swift. This trend changes with the introduction of a surfactant in the water solution.
A 0.5 mM SDS solution has decreased the zeta potentials for the TiO2 nanoparticles as seen in Figure 7, which clearly shows a comparison of P-25 in both the SDS and water solutions and the fact that the IEP has decreased a little bit but that the SDS curve has a smaller slope. The same result was true for the shape-controlled TiO2 nanoparticles, which is clearly seen in Figure 8-10. The available anions and cations from the SDS are shown to have altered the charge of the double layer and created a new and smaller IEP for the shape-controlled TiO2 nanoparticles.
Lower zeta potential is obtained for TiO2 nanoparticles in SDS solution than in water. This can be explained by the semi-micelle model [14]. At high pH, TiO2 nanoparticles show electro-negativity. SDS is adsorbed on the TiO2 nanoparticle surface through non-polar physical adsorption. The adsorption volume is small because of the repulsion between the particles.
When SDS concentration increases, SDS micelles will form on the TiO2 nanoparticle surface, and adsorption volumes increase dramatically in the micelle forming stage. Zeta potential becomes more negative because of the adsorption of surfactant SDS micelles. Repulsion of the surfactant prevents the nanoparticles from flocculation and sedimentation which is the basis for the electrostatic stability of nanoparticles in aqueous suspension.
The zeta potential of TiO2 nanoparticles in an SDS solution of a different concentration is shown in Figure 10. Zeta potential became more negative when SDS concentration increased. Zeta potential became stable when SDS solution reached 1.5mM. Nanoparticles shape controlled by DBS had lower zeta potential than the nanoparticles shape controlled by cellulose in SDS solutions.
Figure 7, Comparison of zeta potential of P-25 in water and SDS solution (SDS conc. 0.5mM)
Figure 8, Zeta potential of cellulose shaped TiO2 nanoparticles in water and SDS solution (SDS conc. 0.5mM)
Figure 9, Zeta potential of DBS shaped TiO2 nanoparticles in water and SDS solution (SDS conc. 0.5mM)
Figure 10, Zeta potential of TiO2 nanoparticles in SDS solutions
Conclusion:
The TiO2 synthesized with different surfactants demonstrate different behaviours, such as the colloidal becoming neutral at a lower pH, where the IEP was lower than pure TiO2 nanoparticles without shape-controlling and P-25, but with the zeta potential remaining positive for a longer range of pH’s than for the other TiO2 synthesized with TiCl4.
The effect of SDS on the zeta potential of TiO2 nanoparticles was also investigated; TiO2 nanoparticles became more negatively charged in SDS solutions and the addition of SDS into the aqueous solution had little effect on the IEP of P-25.
It is therefore concluded that zeta potential can be manipulated during the synthesis process to change the shape and crystal structure of a colloidal in order that the maximization of the desired physical properties can be achieved
References:
[1] Bryce P. Nelson, Roberto Candal and Marc A. Anderson, Langmuir, 2000, 16, 6094-6101.
[2] Shengcong Liufu, Hanning Xiao and Yuping Li, Journal of Colloid and interface Science, 2005, 281, 155-163.
[3] Marye Anne Fox, Maria T. Dulay, Chem. Rev., 1993, 93, 341-357.
[4] Guanli Xu, Jingjie Zhang and Guangzhi Song, J. Dispersion Science and Technology, 2003, 24(3), 527-535.
[5] Masahiro Miyauchi, Ayako Ikezawa and Kazuhito Hashimoto, Phys. Chem. Chem. Phys., 2004, 6, 865-870.
[6] F. Molino, J. M. Barthez and J. Marignan, Physical Review E, 1996, 53(1), 921-925.
[7] Sato T., Kohnosu S, Colloid Surface. A, 1994, 88, 197-205.
[8] N. Mandzy, E. Grulke and T. Druffel, Powder Technology, 2005, 16, 121-126
[9] D. Gumy, C. Morais and P. Bowen, Applied Catalysis B: Environmental 2006, 63, 76–84.
[10] Palarcik J., Jandera, J. and Svoboda, L., CHISA 2004 – 16th International Congress of Chemical and Process Engineering, 2004, 8133-8145.
[11] S. Lebrette, C. Pagnoux, Journal of Colloid and Interface Science, 2004, 280(2), 400-408.
[12] Vladimiros Nikolakis, Current Opinion in Colloid & Interface Science, 2005, 10, 203-210.
[13] Jacques Moser, Swarnalatha Punchihewa and Pierre P. Infelta, Langmuir, 1991, 7, 3012-3018.
[14] Imae T., Muto K., Ikeda S., Coll. Ploym. Sci., 1991(269), 437.
[15] T. Yoko, K. Kamiya, A. Yusa, Journal of Non-crystal Solids, 1998, 100, 483-486.
[16] J. J. Sene, W. A. Zeltner, M. A. Anderson, J. Phys. Chem. B, 2003, 107, 1597-1600.
[17] G. D. Parfitt, Prog. Surf. Membr. Sci., 1976, 11, 81.
[18] Carp O., Huisman C. L, and Reller, A., Progress in Solid State Chemistry, 2004, 32(1-2), 33-177
[19] Croll, S. Progress in Organic Coatings, 2002, 44, 131–146