The Motor Controller
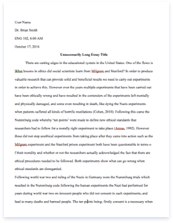
- Pages: 29
- Word count: 7042
- Category: Control General Motors
A limited time offer! Get a custom sample essay written according to your requirements urgent 3h delivery guaranteed
Order Now1 INTRODUCTION
Motor Controller is a device or group of devices that serves to govern in some predetermined manner the performance of an electric motor. A motor controller might include a manual or automatic means for starting and stopping the motor, selecting forward or reverse rotation, selecting and regulating the speed, regulating or limiting the torque, and protecting against overloads and faults. Motor controllers can be manually, remotely or automatically operated. They may include only the means for starting and stopping the motor or they may include other functions. An electric motor controller can be classified by the type of motor it is to drive such as permanent magnet, servo, series, separately excited, and alternating current. A motor controller is connected to a power source such as a battery pack or power supply, and control circuitry in the form of analog or digital input signals. DC motor controller DC motor control circuit using NE555
1.1 DESCRIPTION.
A simple DC motor controller circuit using NE555 is shown here. Many DC motor speed control circuits have been published here but this is the first one using NE555 timer IC. In addition to controlling the motors speed its direction of rotation can be also changed using this circuit. A PWM circuit based on timer NE555 is the heart of this circuit. NE555 is wired as an astable multivibrator whose duty cycle can be adjusted by varying the POT R1. The output of IC1 is coupled to the base of transistor Q1 which drives the motor according to the PWM signal available at its base. Higher the duty cycle the average voltage across motor will be high which results in higher motor speed and vice versa. Change of DC motor direction is attained using the DPDT switch S1 which on application just toggles the polarity applied to the motor. 1.2 BLOCK DIAGRAM DC MOTOR SPEED CONTROL.
CHAPTER 2
PRINTED CIRCUIT BOARD
2 PRINTED CIRCUIT BOARD
A printed circuit board, or PCB, is used to mechanically support and electrically connect electronic components using conductive pathways, tracks or signal traces etched from copper sheets laminated onto a non-conductive substrate. When the board has only copper tracks and features, and no circuit elements such as capacitors, resistors or active devices have been manufactured into the actual substrate of the board, it is more correctly referred to as printed wiring board (PWB) or etched wiring board. Use of the term PWB or printed wiring board although more accurate and distinct from what would be known as a true printed circuit board, has generally fallen by the wayside for many people as the distinction between circuit and wiring has become blurred.
Today printed wiring (circuit) boards are used in virtually all but the simplest commercially produced electronic devices, and allow fully automated assembly processes that were not possible or practical in earlier era tag type circuit assembly processes. A PCB populated with electronic components is called a printed circuit assembly (PCA), printed circuit board assembly or PCB Assembly (PCBA). In informal use the term “PCB” is used both for bare and assembled boards, the context clarifying the meaning. Alternatives to PCBs include wire wrap and point-to-point construction. PCBs must initially be designed and laid out, but become cheaper, faster to make, and potentially more reliable for high-volume production since production and soldering of PCBs can be automated. Much of the electronics industry’s PCB design, assembly, and quality control needs are set by standards published by the IPC organization.
1.1 MANUFACTURING
Materials
Excluding exotic products using special materials or processes, all printed circuit boards manufactured today can be built using the following four items which are usually purchased from manufacturers: 1. Laminates
2. Copper-clad laminates
3. Resin impregnated B-stage cloth (Pre-preg)
4. Copper foil
Laminates
Laminates are manufactured by curing under pressure and temperature layers of cloth or paper with thermoset resin to form an integral final piece of uniform thickness. The size can be up to 4 by 8 feet (1.2 by 2.4 m) in width and length. Varying cloth weaves (threads per inch or cm), cloth thickness, and resin percentage are used to achieve the desired final thickness and dielectric characteristics. The cloth or fiber material used, resin material, and the cloth to resin ratio determine the laminate’s type designation (FR-4, CEM-1, G-10, etc.) and therefore the characteristics of the laminate produced. Important characteristics are the level to which the laminate is fire retardant, the dielectric constant (er), the loss factor (tĪ“), the tensile strength, the shear strength, the glass transition temperature (Tg), and the Z-axis expansion coefficient (how much the thickness changes with temperature). FR-4 is by far the most common material used today. The board with copper on it is called “copper-clad laminate”. Copper foil thickness can be specified in ounces per square foot or micrometres. One ounce per square foot is 1.344 mils or 34 micrometres. 2.2 PATTERNING (ETCHING)
The majority of printed circuit boards today are made from purchased laminate material with copper already applied to both sides. The unwanted copper is removed by various methods leaving only the desired copper traces, this is called subtractive. In an additive method, traces are electroplated onto a bare substrate using a complex process with many steps. The advantage of the additive method is less pollution of the environment. The method chosen for PCB manufacture depends on the desired number of boards to be produced. Double-sided boards or multi-layer boards use plated-through holes, called vias, to connect traces on different layers of the PWB.
* The two processing methods used to produce a double-sided PWB with plated through holes. * Subtractive methods, that remove copper from an entirely copper-coated board, used for the production of printed circuit boards:
FIGURE 2.1 1. Silk screen printing uses etch-resistant inks to protect the copper foil. Subsequent etching removes the unwanted copper. Alternatively, the ink may be conductive, printed on a blank (non-conductive) board. The latter technique is also used in the manufacture of hybrid circuits. 2. Photoengraving uses a photomask and developer to selectively remove a photoresist coating. The remaining photoresist protects the copper foil. Subsequent etching removes the unwanted copper. The photomask is usually prepared with a photoplotter from data produced by a technician using CAM, or computer-aided manufacturing software. Laser-printed transparencies are typically employed for phototools; however, direct laser imaging techniques are being employed to replace phototools for high-resolution requirements. 3. PCB milling uses a two or three-axis mechanical milling system to mill away the copper foil from the substrate. A PCB milling machine (referred to as a ‘PCB Prototyper’) operates in a similar way to a plotter, receiving commands from the host software that control the position of the milling head in the x, y, and (if relevant) z axis. Data to drive the Prototyper is extracted from files generated in PCB design software and stored in HPGL or Gerber file format. Additive processes
Additive processes adds desired copper traces to an insulating substrate. In the full additive process the bare laminate is covered with a photosensitive film which is imaged (exposed to light though a mask and then developed which removes the unexposed film). The exposed areas are sensitized in a chemical bath, usually containing palladium and similar to that used for through hole plating which makes the exposed area capable of bonding metal ions. The laminate is then plated with copper in the sensitized areas. When the mask is stripped, you have a finished PCB. The most common is the “semi-additive” process: the unpatterned board has a thin layer of copper already on it.
A reverse mask is then applied. (Unlike a subtractive process mask, this mask exposes those parts of the substrate that will eventually become the traces.) Additional copper is then plated onto the board in the unmasked areas; copper may be plated to any desired weight. Tin-lead or other surface plating are then applied. The mask is stripped away and a brief etching step removes the now-exposed bare original copper laminate from the board, isolating the individual traces. Some single-sided boards which have plated-through holes are made in this way. General Electric made consumer radio sets in the late 1960s using additive boards. In 1977 Dr.J.F.Mansfeld presented the PD-R process (Physical Development by Reduction) in a speech at the Hotel International Zurich. The additive process is commonly used for multi-layer boards as it facilitates the plating-through of the holes to produce conductive vias in the circuit board.
2.3 PCB DESIGN
Printed circuit board artwork generation was initially a fully manual process done on clear mylar sheets at a scale of usually 2 or 4 times the desired size. The schematic diagram was first converted into a layout of components pin pads, then traces were routed to provide the required interconnections. Pre-printed non-reproducing mylar grids assisted in layout, and rub-on dry transfers of common arrangements of circuit elements (pads, contact fingers, integrated circuit profiles, and so on) helped standardize the layout. Traces between devices were made with self-adhesive tape. The finished layout “artwork” was then photographically reproduced on the resist layers of the blank coated copper-clad boards. Modern practice is less labor intensive since computers can automatically perform many of the layout steps. The general progression for a commercial printed circuit board design would include:
1. Schematic capture through an Electronic design automation tool. 2. Card dimensions and template are decided based on required circuitry and case of the PCB. Determine the fixed components and heat sinks required. 3. Deciding stack layers of the PCB. 1 to 12 layers or more depending on design complexity. Ground plane and power plane are decided. Signal planes where signals are routed are in top layer as well as internal layers. 4. Line impedance determination using dielectric layer thickness, routing copper thickness and trace-width. Trace separation also taken into account in case of differential signals. Microstrip, stripline or dual stripline can be used to route signals. 5. Placement of the components. Thermal considerations and geometry are taken into account. Vias and lands are marked. 6. Routing the signal tracs. For optimal EMI performance high frequency signals are routed in internal layers between power or ground planes as power planes behave as ground for AC. 7. Gerber file generation for manufacturing.
In the design of the PCB artwork, a power plane is the counterpart to the ground plane and behaves as an AC signal ground, while providing DC voltage for powering circuits mounted on the PCB. In electronic design automation (EDA) design tools, power planes (and ground planes) are usually drawn automatically as a negative layer, with clearances or connections to the plane created automatically.
2.4 PCB LAYOUT
The connections on a PCB should be identical to its corresponding circuit diagram, but while the circuit diagram is arranged to be readable, the PCB layout is arranged to be functional, so there is rarely any visible correlation.
PCB layout can be performed manually (using CAD) or in combination with an Autorouter. The best results are usually still achieved using atleast some manual routing – simply because the design engineer has a far better judgement of how to arrange circuitry. Surprisingly, many autorouted boards are often completely illogical in their track routing – the program has optimised the connections, and sacrificed any small amount of order that may have been put in place by manual routing. Generally autorouted boards are somewhat harder for a technician to repair or debug, for this reason. Historically, PCBs used to be laid out by using stick on paper shapes and tape on mylar drafting film, – that really WAS manual routing!
CHAPTER 3 SOLDERING
SOLDERING
Soldering is a process in which two or more metal items are joined together by melting and flowing a filler metal (solder) into the joint, the filler metal having a lower melting point than the work piece. Soldering differs from welding in that soldering does not involve melting the work pieces. In brazing, the filler metal melts at a higher temperature, but the workpiece metal does not melt. Formerly nearly all solders contained lead, but environmental concerns have increasingly dictated use of lead-free alloys for electronics and plumbing purposes. |
3.1 APPLICATIONS
Soldering is used in plumbing, in electronics and metalwork from flashing to jewelry. Soldering provides reasonably permanent but reversible connections between copper pipes in plumbing systems as well as joints in sheet metal objects such as food cans, roof flashing, rain gutters and automobile radiators. Jewelry components, machine tools and some refrigeration and plumbing components are often assembled and repaired by the higher temperature silver soldering process. Small mechanical parts are often soldered or brazed as well. Soldering is also used to join lead came and coppe rfoil in stained glass work. It can also be used as a semi-permanent patch for a leak in a container or cooking vessel. Electronic soldering connects electrical wiring and electronic components to printed circuit boards (PCBs). 3.2 SOLDERS
Soldering filler materials are available in many different alloys for differing applications. In electronics assembly, the eutectic alloy of 63% tin and 37% lead (or 60/40, which is almost identical in melting point) has been the alloy of choice. Other alloys are used for plumbing, mechanical assembly, and other applications. Some examples of soft-solder are tin-lead for general purposes, tin-zinc for joining aluminium , lead-silver for strength at higher than room temperature, cadmium-silver for strength at high temperatures, zinc-aluminium for aluminium and corrosion resistance, and tin-silver and tin-bismuth for electronics. Common solder formulations based on tin and lead are listed below. The fraction represent percentage of tin first, then lead, totaling 100%: * 63/37: melts at 183 °C (361 °F) (eutectic: the only mixture that melts at a point, instead of over a range) * 60/40: melts between 183ā190 °C (361ā374 °F)
* 50/50: melts between 185ā215 °C (365ā419 °F)
For environmental reasons (and the introduction of regulations such as the European RoHS (Restriction of Hazardous Substances Directive)), lead-free solders are becoming more widely used. They are also suggested anywhere young children may come into contact with (since young children are likely to place things into their mouths), or for outdoor use where rain and other precipitation may wash the lead into the groundwater. Unfortunately, most lead-free solders are not eutectic formulations, melting at around 250 °C (482 °F), making it more difficult to create reliable joints with them. Other common solders include low-temperature formulations (often containing bismuth), which are often used to join previously-soldered assemblies without un-soldering earlier connections, and high-temperature formulations (usually containing silver) which are used for high-temperature operation or for first assembly of items which must not become unsoldered during subsequent operations. Alloying silver with other metals changes the melting point, adhesion and wetting characteristics, and tensile strength. Of all the brazing alloys, silver solders have the greatest strength and the broadest applications. Specialty alloys are available with properties such as higher strength, the ability to solder aluminum, better electrical conductivity, and higher corrosion resistance. 3.3 SOLDERABILITY
The solderability of a substrate is a measure of the ease with which a soldered joint can be made to that material. 3.4 DESOLDERING AND RESOLDERING
Used solder contains some of the dissolved base metals and is unsuitable for reuse in making new joints. Once the solder’s capacity for the base metal has been achieved it will no longer properly bond with the base metal, usually resulting in a brittle cold solder joint with a crystalline appearance. It is good practice to remove solder from a joint prior to resolderingādesoldering braids or vacuum desoldering equipment (solder suckers) can be used. Desoldering wicks contain plenty of flux that will lift the contamination from the copper trace and any device leads that are present. This will leave a bright, shiny, clean junction to be resoldered. The lower melting point of solder means it can be melted away from the base metal, leaving it mostly intact, though the outer layer will be “tinned” with solder. Flux will remain which can easily be removed by abrasive or chemical processes. This tinned layer will allow solder to flow into a new joint, resulting in a new joint, as well as making the new solder flow very quickly and easily.
CHAPTER 4
PULSE-WIDTH MODULATION
PULSE-WIDTH MODULATION (PWM)
Pulse-width modulation (PWM) is a modulation technique that conforms the width of the pulse, formally the pulse duration, based on a modulator signal information. Although this modulation technique can be used to encode information for transmission, its main use is to allow the control of the power supplied to electrical devices, especially to inertial loads such as motors. The average value of voltage (and current) fed to the load is controlled by turning the switch between supply and load on and off at a fast pace. The longer the switch is on compared to the off periods, the higher the power supplied to the load is. The PWM switching frequency has to be much faster than what would affect the load, which is to say the device that uses the power. Typically switching have to be done several times a minute in an electric stove, 120 Hz in a lamp dimmer, from few kilohertz (kHz) to tens of kHz for a motor drive and well into the tens or hundreds of kHz in audio amplifiers and computer power supplies.
The term duty cycle describes the proportion of ‘on’ time to the regular interval or ‘period’ of time; a low duty cycle corresponds to low power, because the power is off for most of the time. Duty cycle is expressed in percent, 100% being fully on. The main advantage of PWM is that power loss in the switching devices is very low. When a switch is off there is practically no current, and when it is on, there is almost no voltage drop across the switch. Power loss, being the product of voltage and current, is thus in both cases close to zero. PWM also works well with digital controls, which, because of their on/off nature, can easily set the needed duty cycle. PWM has also been used in certain communication systems where its duty cycle has been used to convey information over a communications channel. An example of PWM in an AC motor drive: the phase-to-phase voltage (blue) is modulated as a series of pulses that results in a sine-like flux density waveform (red) in the magnetic circuit of the motor. The smoothness of the resultant waveform can be controlled by the width and number of modulated impulses (per given cycle)
FIGURE 4.1
CHAPTER 5
CONSTRUCTION
CONSTRUCTION
First we take a general purpose PCB of minimum size. Then sold the 8-pin NE-555 IC base on it. Now we make upper & lower row of PCB short to make neutral & line.
* From pin no.1 of IC NE555 we sold a wire & connect it through ground. * Pin 2& 6 are short together from which a capacitor of 0.1 micro F is connected to neutral. * Pin 3 is sold with 33 ohm resister & connect to base of npn transistor Q1 i.e. BD139. * Pin 4 & Pin 8 are short and connect to line at which we give supply of + 12V VD & then a resistance R2 of 1K ohm is connect. * At pin 5 another capacitor of 0.01 micro F is sold with other terminal sold to neutral. * Pin 7 is sold with a pair of diode 1N 4001 which is sold with 1K ohm resistor R2 as shown in circuit diagram of DC motor control. * Both the diode and pin 6 is sold with three terminal of voltage regulator R1. * Now, all the connection with IC NE555 has been done.
Now place a npn transistor Q1 named BD 139. The base of transistor is connect to pin 3 of NE555 IC via 33 ohm resistance & collector is sold with neutral. Two wires are sold with 3rd pin i.e. emitter of BD 139.One goes to supply Vm via diode D3 1N4007 & another connect to DPDT switch.
One of the terminal of DPDT switch is connect to Vm. Finally we connect a DC motor with DPDT switch.
CHAPTER 6
CIRCUIT DIAGRAM OF DC MOTOR CONTROL
CIRCUIT DIAGRAM OF DC MOTOR CONTROL
FIGURE 6.1
* The circuit can be assembled on a Vero board or PCB.
* Use 12V DC for powering the IC.
* Vm is the power supply for motor and its value depends on the motors voltage rating. Any way maximum Vceo for BD139 is 80V and so Vm should not be exceeded 80 volts. * Maximum collector current BD139 can handle is 1.5A and so do not use a motor that consumes more than 1.5 amperes of current. * A heat sink is necessary for BD139.
6.1 WORKING
A simple DC motor controller circuit with NE555 is shown here. several DC motor speed control circuits are revealed here however this can be the first one using NE555 timer IC. additionally to controlling the motors speed its direction of rotation will be also modified using this circuit.
A PWM circuit primarily based on timer NE555 is that the heart of this circuit. NE555 is wired as an astable multivibrator whose duty cycle will be adjusted by varying the POT R1. The output of IC1 is coupled to the base of transistor Q1 that drives the motor according to the PWM signal available at its base. Higher the duty cycle the typical voltage across motor will be high which ends in higher motor speed and vice versa. modification of DC motor direction is attained using the DPDT switch S1 that on application simply toggles the polarity applied to the motor.
FIGURE 6.2
The working of IC NE555 was very important to know since it was widely used in many applications. The IC 555 was widely used for producing delays, oscillators and much more. The working of IC 555 was based on the internal components as you can see in the diagram above .It consists of Comparator, Flip flop and transistors which makes this IC work. Now let see the working of this IC.
Here in this IC one Comparator on the right side has a threshold pin 6 and a control pin 5.The control voltage was internally wired which equals the control voltage as +2/3 Vcc. Output of this comparator is applied to set S input of the Flip flop. Whenever the threshold voltage exceeds the control voltage, comparator will set the flip flop and its output will be high. A high output from the flip flop saturates the discharge transistor and it leads to discharging of the capacitor connected externally to the pin 7.The complementary signal out of the flip flop goes into the pin 3 as output. At this stage the output obtained at the pin 3 of the IC will be low. The above conditions will exist until the comparator 2 on left top hand triggers the flip flop. Now even if the voltage at the threshold input falls below 2/3 Vcc, that is comparator 1 cannot cause the flip flop to change again.
To change the output of Flip flop to low the voltage at the trigger input must fall below +1/3 Vcc. When this occurs comparator 2 triggers the flip flop forcing its output low. The low output from the flip flop turns the discharge transistor off and forces the power amplifier to output a high signal. These conditions will continue independent of the voltage on the trigger input.
From the above we can understand basic two things. For getting low output from the timer 555 the voltage on the threshold must drop below +2/3 Vcc and it also turn the discharge transistor ON. To force the output from the timer high the voltage on the trigger must drop below +1/3 Vcc. This also turns the discharge transistor OFF.
A voltage may be applied to the control input to change levels at which the switching occurs. When not in use it is advised to connect a capacitor of 0.01nF between pin 5 and ground to prevent the noise coupled onto the pin to prevent false triggering. The pin 4 was used for the reset purpose. Connecting this pin to logic low will place a high on the output of the flip flop. The discharge transistor will go on and the power amplifier will output a low. This condition will continue until the reset is taken high. This is mainly used for synchronization or resetting of the circuits operation. When not in use it should be connected to the +Vcc.
CHAPTER 7 COMPONENT DESCRIPTION
COMPONENT DESCRIPTION
7.1 DC Motor
A DC motor is a mechanically commutated electric motor powered from direct current (DC). The stator is stationary in space by definition and therefore its current. The current in the rotor is switched by the commutator to also be stationary in space. This is how the relative angle between the stator and rotor magnetic flux is maintained near 90 degrees, which generates the maximum torque. DC motors have a rotating armature winding (winding in which a voltage is induced) but non-rotating armature magnetic field and a static field winding (winding that produce the main magnetic flux) or permanent magnet. Different connections of the field and armature winding provide different inherent speed/torque regulation characteristics. The speed of a DC motor can be controlled by changing the voltage applied to the armature or by changing the field current. The introduction of variable resistance in the armature circuit or field circuit allowed speed control. Modern DC motors are often controlled by power electronics systems called DC drives. Working or Operating Principle of DC Motor
A DC Motor in simple words is a device that converts direct current(electrical energy) into mechanical energy. Itās of vital importance for the industry today, and is equally important for engineers to look into the working principle of DC motor. In order to understand the operating principle of dc motor we need to first look into its constructional feature. FIGURE 7.1.1
The very basic construction of a dc motor contains a current carrying armature which is connected to the supply end through commutator segments and brushes and placed within the north south poles of a permanent or an electro-magnet as shown in the diagram below. Flemingās left hand rule says that if we extend the index finger, middle finger and thumb of our left hand in such a way that the current carrying conductor is placed in a magnetic field (represented by the index finger) is perpendicular to the direction of current (represented by the middle finger), then the conductor experiences a force in the direction (represented by the thumb) mutually perpendicular to both the direction of field and the current in the conductor. For clear understanding the principle of DC motor we have to determine the magnitude of the force, by considering the diagram below. We know that when an infinitely small charge dq is made to flow at a velocity āvā under the influence of an electric field E, and a magnetic field B, then the Lorentz Force dF experienced by the charge is given by:-
FIGURE 7.1.2
dF = dq(E + v X B)
For the operation of dc motor, considering E = 0
ā“ dF = dq v X B
i.e. itās the cross product of dq v and magnetic field B.
or dF = dq (dL/dt) X B [v = dL/dt]
Where dL is the length of the conductor carrying charge q.
or dF = (dq/dt) dL X B
or dF = I dL X B [Since, current I = dq/dt]
or F = IL X B = ILB SinĪø
or F = BIL SinĪø
From the 1st diagram we can see that the construction of a dc motor is such that the direction of electric current through the armature conductor at all instance is perpendicular to the field. Hence the force acts on the armature conductor in the direction perpendicular to the both uniform field and current is constant.
i.e. θ = 90°
So if we take the current in the left hand side of the armature conductor to be I, and current at right hand side of the armature conductor to be ā I, because they are flowing in the opposite direction with respect to each other. Then the force on the left hand side armature conductor, Fl = BIL Sin90° = BIL Similarly force on the right hand side conductor Fr = B( ā I)L.Sin90° = ā BIL
ā“ we can see that at that position the force on either side is equal in magnitude but opposite in direction. And since the two conductors are separated by some distance w = width of the armature turn, the two opposite forces produces a rotational force or a torque that results in the rotation of the armature conductor.Now letās examine the expression of torque when the armature turn crate an angle of α with its initial position. The torque produced is given by
Torque = force, tangential to the direction of armature rotation X distance.
or Ļ = Fcosα.w
or Ļ = BIL w cosα
Where α is the angle between the plane of the armature turn and the plane of reference or the initial position of the armature which is here along the direction of magnetic field.The presence of the term cosα in the torque equation very well signifies that unlike force the torque at all position is not the same. It in fact varies with the variation of the angle α.  FIGURE 7.1.3
Step 1:
Initially considering the armature is in its starting point or reference position where the angle α = 0.
ā“ Ļ = BIL w cos0 = BILw
Since α = 0, the term cos α = 1, or the maximum value, hence torque at this
position is maximum given by Ļ = BILw. This high starting torque helps in overcoming the initial inertia of rest of the armature and sets it into rotation.
FIGURE 7.1.4
Step 2
Once the armature is set in motion, the angle α between the actual position of the armature and its reference initial position goes on increasing in the path of its rotation until it becomes 90° from its initial position. Consequently the term cosα decreases and also the value of torque.
The torque in this case is given by Ļ = BILwcosα which is less than BIL w when α is greater than 0°
FIGURE 7.1.5
Step 3:
In the path of the rotation of the armature a point is reached where the actual position of the rotor is exactly perpendicular to its initial position, i.e. α = 90°, and as a result the term cosα = 0.
The torque acting on the conductor at this position is given by.
Ļ = BILwcos90° = 0
FIGURE 7.1.6
i.e. virtually no rotating torque acts on the armature at this instance. But still the armature does not come to a standstill, this is because of the fact that the operation of dc motor has been engineered in such a way that the inertia of motion at this point is just enough to overcome this point of null torque. Once the rotor crosses over this position the angle between the actual position of the armature and the initial plane again decreases and torque starts acting on it again.
* Specifications:
* Motor Speed Controlled via a potentiometer.
* For DC motors 12 to 32 Volts DC @ 5 Amps as constructed. The IRFZ44 Mosfets can handle up to a maximum 49Amps, but PCB trace capacity would have to be beefed up with some hookup wire (underneath the PCB) soldered between the MOFSET pins and the screw terminal blocks. If you are running beyond 5 Amps and the MOFSETS are getting hot, bigger heatsinks should be used. You can also dissipate heat with a cooling fan directed over the heat sinks. * 1000 RPM.
* Requires operating voltage of 6 – 32 VDC.
7.2 NE-555
GENERAL DESCRIPTION
* The NE555 is a highly stable device for generating accurate time delays or oscillation. Additional terminals are provided for triggering or resetting if desired. In the time delay mode of operation, the time is precisely controlled by one external resistor and capacitor. For astable operation as an oscillator, the free running frequency and duty cycle are accurately controlled with two external resistors and one capacitor. The circuit may be triggered and reset on falling waveforms, and the output circuit can source or sink up to 200mA or drive TTL circuits.
PIN DESCRIPTION
FIGURE 7.2.1
Pin Description: Pin No| Function| Name|
1| Ground (0V)| Ground|
2| Voltage below 1/3 Vcc to trigger the pulse| Trigger|
3| Pulsating output| Output|
4| Active low; interrupts the timing interval at Output| Reset| 5| Provides access to the internal voltage divider; default 2/3 Vcc| Control Voltage| 6| The pulse ends when the voltage is greater than Control| Threshold| 7| Open collector output; to discharge the capacitor|
Discharge| 8| Supply voltage; 5V (4.5V – 16 V)| Vcc|
TABLE 7.2.1
FEATURES
* Direct replacement for SE555/NE555
* Timing from microseconds through hours
* Operates in both astable and monostable modes
* Adjustable duty cycle
* Output can source or sink 200 mA
* Output and supply TTL compatible
* Normally on and normally off output
APPLICATIONS
* Precision timing
* Pulse generation
* Sequential timing
* Time delay generation
* Pulse width modulation
* Pulse position modulation
* Linear ramp generator
7.3 SWITCH
Technical Defination :
⢠Pole – number of switch contact sets.
⢠Throw – number of conducting positions, single or double. ⢠Way – number of conducting positions, three or more.
⢠Momentary (non latching) – switch returns to its normal position when released. ⢠Open – off position, contacts not conducting.
⢠Closed – on position
DPDT Switches
DPDT stands for Double Pole Double Throw. The double pole is for a dual switched output and the double throw is for the on or off conditions. DPDT consists of two separate switches that operate at the same time, each one with normally open and normally closed contact through a common connector. Each of the two contacts on the switch can be routed in different ways, depending on the position of the switch. An example of which is a mini-toggle switch or a switch using a push or pull contact.
FIGURE 7.3.1
Features
DPDT switches commonly use polarity reversal. That is why some variations of the DPDT switch, such as the cross-over switches, are internally wired for that purpose. The cross-over switches have only four terminals or connections, as opposed to six that you see on DPDT. There are two connections used for the outputs and the other two for the inputs. The switch then selects either normal or reversed polarity when connected to any DC source such as the battery. A DPDT Relay has a single coil with two arms that move simultaneously. Inside of the DPDT relay, there are two separate SPDT (Single Pole Double Throw) switch mechanisms. These are being used for signal switching applications more often than not, but can also be found in high power switching applications. Function
A DPDT can be used on any application that requires a NO (Normally Open) and NC (Normally Closed) wiring system, an example of which is Railroad Modelling or Model Railroading, a hobby that makes use of small scaled trains and railways, even small scale cars and bridges. The NC allows for the system to be “on” at all times while NO allows for another piece to be turned “on” or activated through the relay. DPDT can be used in such cases for block control due to the ease and flexibility of wiring, as well as, coil voltage and coil amperage capabilities. In block controls, it is important to be able to take the higher power due to multiple turn ons and turn offs on the system. Block control allows the relay coil to be triggered remotely, while another relay is controlling another block. It is important to assess the priority needs when employing the block control, which accessory or application would need to be prioritized that would be turned on while the other one is turned off to make way for it.
7.4 BD 139 TRANSISTOR
DESCRIPTION
BD139, BD140 are epitaxial planar transistors which are mounted in the SOT-32 plastic package. They are designed for audio amplifiers and drivers utilizing complementary or quasi-complementary circuits. The NPN types are the BD135 and BD139, and the complementary PNP types are the BD136 and BD140. They are used in Medium Power Linear and Switching Applications. BD135, BD137 and BD139 are complement to BD136, BD138 and BD140 respectively.
FIGURE 7.4.1
FIGURE 7.4.2
* FEATURES
High current (max. 1.5 A)
Ā· Low voltage (max. 80 V).
* APPLICATIONS
ļ
ļ Driver stages in hi-fi amplifiers and television circuits
7.5 CAPACITORS
A capacitor (originally known as condenser) is a passive two-terminal electrical component used to store energy in an electric field. The forms of practical capacitors vary widely, but all contain at least two electrical conductors separated by a dielectric (insulator); for example, one common construction consists of metal foils separated by a thin layer of insulating film. Capacitors are widely used as parts of electrical circuits in many common electrical devices. When there is a potential difference (voltage) across the conductors, a static electric field develops across the dielectric, causing positive charge to collect on one plate and negative charge on the other plate. Energy is stored in the electrostatic field. An ideal capacitor is characterized by a single constant value, capacitance, measured in farads. This is the ratio of the electric charge on each conductor to the potential difference between them.
The capacitance is greatest when there is a narrow separation between large areas of conductor, hence capacitor conductors are often called plates, referring to an early means of construction. In practice, the dielectric between the plates passes a small amount of leakage current and also has an electric field strength limit, resulting in a breakdown voltage, while the conductors and leads introduce an undesired inductance and resistance. Capacitors are widely used in electronic circuits for blocking direct current while allowing alternating current to pass, in filter networks, for smoothing the output of power supplies, in the resonant circuits that tune radios to particular frequencies, in electric power transmission systems for stabilizing voltage and power flow, and for many other purposes.
FIGURE 7.5.1
7.6 RESISTERS
FIGURE 7.6.1
A resistor is a passive two-terminal electrical component that implements electrical resistance as a circuit element. The current through a resistor is in direct proportion to the voltage across the resistor’s terminals. This relationship is represented by Ohm’s law:
Where I is the current through the conductor in units of amperes, V is the potential difference measured across the conductor in units of volts, and R is the resistance of the conductor in units of ohms. The ratio of the voltage applied across a resistor’s terminals to the intensity of current in the circuit is called its resistance, and this can be assumed to be a constant (independent of the voltage) for ordinary resistors working within their ratings. Resistors are common elements of electrical networks and electronic circuits and are ubiquitous in electronic equipment. Practical resistors can be made of various compounds and films, as well as resistance wire (wire made of a high-resistivity alloy, such as nickel-chrome). Resistors are also implemented within integrated circuits, particularly analog devices, and can also be integrated into hybrid and printed circuits. The electrical functionality of a resistor is specified by its resistance: common commercial resistors are manufactured over a range of more than nine orders of magnitude. When specifying that resistance in an electronic design, the required precision of the resistance may require attention to the manufacturing tolerance of the chosen resistor, according to its specific application. The temperature coefficient of the resistance may also be of concern in some precision applications.
CHAPTER 8
APPLICATION
APPLICATION
Every electric motor has to have some sort of controller. The motor controller will have differing features and complexity depending on the task that the motor will be performing. The simplest case is a switch to connect a motor to a power source, such as in small appliances or power tools. The switch may be manually operated or may be a relay or contactor connected to some form of sensor to automatically start and stop the motor. The switch may have several positions to select different connections of the motor. This may allow reduced-voltage starting of the motor, reversing control or selection of multiple speeds.
Overload and over current protection may be omitted in very small motor controllers, which rely on the supplying circuit to have over current protection. Small motors may have built-in overload devices to automatically open the circuit on overload. Larger motors have a protective overload relay or temperature sensing relay included in the controller and fuses or circuit breakers for over current protection. An automatic motor controller may also include limit switches or other devices to protect the driven machinery. More complex motor controllers may be used to accurately control the speed and torque of the connected motor (or motors) and may be part of closed loop control systems for precise positioning of a driven machine. For example, a numerically controlled lathe will accurately position the cutting tool according to a preprogrammed profile and compensate for varying load conditions and perturbing forces to maintain tool position.
* CHRONOLOGY
The following steps have been followed in carrying out the project: * Study the books on relevant topic.
* Understand the working of circuit.
* Prepare the circuit diagram.
* Prepare the list of component along with their specification. Estimate the cost and procure them after carrying out market survey.
* Plan and prepare PCB for mounting all components.
* Fix the components on PCB and solder them.
* Test the circuit for the desired performance.
* Trace and rectify fault if any.
* Give good finish to the unit.
* Prepare the project report.