Microporosity and Surface Functionality of Activated Carbon
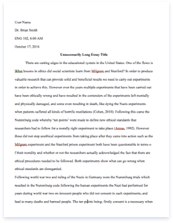
- Pages: 23
- Word count: 5536
- Category: Carbon
A limited time offer! Get a custom sample essay written according to your requirements urgent 3h delivery guaranteed
Order Now
Abstract
Activated carbons have been prepared from jute stick by both chemical and physical activation methods using ZnCl2 and steam, respectively. The activated carbons were characterized by evaluating surface area, iodine number, pore size distribution, surface functional groups and surface textural properties. Based on the analysis, the activated carbon prepared by chemical activation method, (ACC) featured micropore structure, while the activated carbon prepared by physical activation, (ACS) largely featured macropore structure. The BET surface area of ACC and ACS was 2300 m2/g and 730 m2/g, respectively. The FT-IR spectra revealed that a significant number of organic functional groups are randomly distributed on the large surface area of ACC, meanwhile it was very limited for ACS. The microporosity along with surface functional groups provided a unique property to ACC to adsorb methylene blue dye, which is a representative basic dye for textile industries, in a large extent compared to ACS. The adsorption of dye using both ACC and ACS was also affected by the adsorption parameters such as adsorption time, temperature and pH. Comparatively higher temperature and pH facilitated dye adsorption significantly, especially for ACC.
Keywords: Activated carbon, Jute stick, Chemical activation, Methylene blue, Adsorption
1. Introduction
Activated carbons (AC) possess high surface area with porous structures and are widely known as efficient adsorbents for both gas and liquid phase adsorptions. The increasing environmental concern significantly increased the applicability of AC for industrial pollutants separation. The effluents from industries, such as textile, leather, paper, ink and cosmetics as well as from the industries that produce dyes are severely contaminated with dyes, pigments, surfactants and many other toxic chemicals. These contaminated effluents ultimately go to the surface water reservoir. The dye contaminated water even in a very low concentration is visible and aesthetically unacceptable. As most dyes are toxic and primarily contaminate surface water, the water biota is the primary victim of dye contamination, and long exposure of dyes in water often causes food chain contamination, resulting in adverse health effect. Hence, it is mandatory to reduce contaminant concentration in effluent bellow acceptable range before being released into the environment by utilizing proper treatment process. Due to the technological advancement, numerous processes have been attempted to remove dyes and other contaminants from effluent in the last few decades.
The most frequently used processes are adsorption [1-4], oxidationâozonation [5], photocatalysis [6], biological treatment [7], coagulationâflocculation [8] and membrane separation [9]. Among the processes, the adsorption is the most versatile and economic due to many advantages. Although the biological treatment for organic compounds removal from water is some extent effective, the removal of organic refractory contaminants has proven to be very ineffective. Even the contaminants are non reactive, the adsorbent can remove contaminants satisfactorily [10-12]. A number of adsorbents are used for dye removal including agricultural wastes, wood materials, industrial wastes and synthetic materials [1]. Activated carbons, which can be produced from agricultural wastes, are known as very effective adsorbents for dye adsorption [13]. The unique adsorption property is developed on the AC surface due to the development of microporosity, large surface area (usually 500 -3000 m2/g and variable characteristics of surface chemistry [14, 15]. These properties of AC can be regulated by regulating the preparation methods and their conditions as well as by selecting the precursor materials. A very selective AC can be prepared with a precise preparation method from a suitable precursor for a specific dye separation.
The adsorption of dye molecules onto the AC surface depends on the pore size distribution, surface functionality as well as the size and shape selectivity of the molecules to be adsorbed. For an optimum adsorption, the molecular size of the adsorbates needs to be quite fitted to the pore size of the AC. The pore size of the AC is classified as micropore (< 2 nm), mesopore (2-5 nm) and macropore (>5 nm) [16]. Both in the gas phase and liquid phase separations, the micro- and mesopores play major role [17, 18]. The macropore structure leads to the smaller surface area of AC as well as the multilayer adsorption is limited in macropore, which attributes to the lower adsorption capacity. Therefore, the pore size and structure of AC need to be optimized for a specific separation. The activated carbon from hard wood is especially used for gas separation and that is from soft wood is used for solution phase separation. The chemical activation using H3PO4, ZnCl2, KOH and K2CO3 is known to produce micropore and mesopore structure in AC [19].
However, the physical activation using steam often produces activated carbon with macropore structure [20]. Activated carbons from the soft cellulosic precursors, especially from agricultural residues are widely used for dye molecule separation from solution [1]. Phenol and many inorganic contaminants such as arsenic and mercury are also potentially environmental hazards and are separated using AC prepared from agricultural residues [21]. The presence of functional groups on AC surface provides polarity, which in turns influence adsorption properties. The IR spectroscopic studies represented that during heat treatment at high temperature to produce AC, most of the reactive functional groups on the surface of biomass are released as H2O, CO2 and many other small molecules, leaving behind quinolic, etheric, phenolic and ketonic functional groups [22].
These oxygen containing surface functional groups provide acidic as well as basic properties depending on the ring structures [23]. The extent of ring condensation during activation has also a role to play to form a wide basal surface of AC, which facilitates the accommodation of dye molecules in adsorption. Some studies showed that during steam activation of char at 700-900oC the structural features of carbon drastically changed due to the condensation of smaller ring systems (3-5 fused rings) to larger ring systems (â„6 fused rings) [24]. The studies also showed that the reactivity of remaining char with oxygen drastically decreased with increasing the contact time of steam with char. However, since the chemical activated carbon is often produced at around 500oC, much lower than steam activation temperature, a significant number of functional groups are assumed to be retained on the surface. The preparation, characterization and utilization of activated carbons for dye separation were the objectives of this study. Jute stick, which is abundantly available agricultural residues in most of the Asian countries, was used as a precursor for activated carbon preparation by chemical and physical activation methods. Both of the activated carbons were characterized and utilized for methylene blue dye separation.
2. Experimental
2. 1. Preparation of feedstock
Jute stick of commonly cultivated varieties, Corchorus capsularis, in Asian countries was collected, washed with distilled water and dried at 105oC for 12 h. The particle size of 1-2 mm was prepared by grinding and sieving of original jute stick. The moisture content of the dried jute stick was found to be approximately 4 wt%. The physical properties, proximate and ultimate analyses of jute sticks have been published elsewhere [25]. The proximate analysis exhibited 76â78 wt% volatile fraction, 21â23 wt% fixed carbon and 0.62 wt% ash yield. The ultimate analysis resulted in 49.79 wt% C, 6.02 wt% H, 41.37 wt% O, 0.19 wt% N, 0.05 wt% Cl and 0.05 wt% S. Zinc chloride (99.0 %, BDH Chemical Co.) was used as an activating agent. Iodine (99.5 %), Sodium thiosulphate (99 %), Potassium iodide (99.5 %), Hydrochloric acid (35 %), Potassium dichromate (99.9 %) were purchased from Loba Chemicals Co., India. Commercial activated carbon (Laboratory Reagent, Thomas Baker Chemicals Ltd.) and Methylene Blue (99.9 %, Aldrich) were used in this investigation.
2.2 Preparation of activated carbon
The preparation of activated carbon from jute stick by chemical activation using ZnCl2 involved three steps: (1) socking of reagent solution by jute stick particles, (2) low temperature (200 oC) carbonization to produce char and (3) high temperature (500 oC) activation of char. About 50 g of dried jute sticks was mixed with ZnCl2 solution and kept for about 15 h at room temperature. The solution was completely socked by the solid mass. The ZnCl2 to jute stick ratio was adjusted to 1:1. The wet solid was then transferred into a stainless steel reactor. The reactor configuration, published elsewhere [26], was 25 cm long with 5 cm internal diameter. The inside temperature of the reactor was controlled by a temperature controller through a thermocouple inserted into the reactor. Before heating the reactor, nitrogen gas was purged for about 10 min at the rate of 200 mL/min to replace the air inside the reactor. Then the reactor was heated to 200 oC at the heating rate of 5 oC/min and was held for at least 15 min at this temperature, while the nitrogen gas was continued to flow. During this operation, the jute stick particles were carbonized and converted to sticky and black semi solid mass.
The temperature was further increased to activation temperature at the heating rate of 5 oC/min. The carbonized product was activated at around 500 oC. The final product was washed with deionised water. For washing, the solid product was mixed with deionised water in a beaker and stirred for 20 min at 60 oC and then the solid mass was separated by means of a vacuum filter. The process was repeated in order to remove ZnCl2 almost completely. To detect the presence of ZnCl2 in the spent water, 2/3 mL of it was taken in a test tube and a few drops of AgNO3 solution was added into it. Appearing of a white precipitate indicated the presence of ZnCl2. Therefore, the washing of the product was repeated until no existence of white precipitate observed in the test tube. Finally, the AC sample was dried at 105oC for 24 h and stored in a desiccator. The activated carbon prepared by chemical activation method was denoted as ACC. From the total weight loss due to the activation of jute stick, the yield and activation burn-off were calculated using the following Equations 1 and 2, respectively: Y (%) = [pic] âŠâŠâŠâŠâŠâŠâŠâŠ.(1)
Y( (%) = [pic]âŠ.âŠâŠâŠâŠâŠ(2)
where Y and Y( are yield of activated carbon and activation burn-off, respectively. M (g) is mass of activated carbon obtained and Mo is initial mass of jute sticks in dry basis. The activated carbon was also prepared by physical activation method using steam under optimum conditions as investigated in our previous work [27]. In this method, 50 g of jute stick was placed in a stainless steel reactor and was heated to 700 oC at the heating rate of 5 oC/min under the nitrogen gas flow of 200 mL/min. When the temperature attained to 700 oC, the steam was started to flow through the char bed into the reactor. The steam flow rate was 75 mg/min. For steam generation, the water was supplied by a Peristaltic Pump into the upper hot zone of the reactor, where the water was suddenly vaporized and the vapour was mixed up with nitrogen gas and went through the char bed. When the steam contacted the char, a gasification reaction took place, releasing CO and CO2 and leaving behind the porous carbon structure. At the end of the process the activated carbon was allowed to cool to room temperature. Finally, the activated carbon was washed with 0.1 M HCl to remove ash and then washed with deionised water to remove residual acid. This activated carbon was denoted as ACS.
2.3Characterization
The ACC and ACS were characterized in terms of specific surface area, iodine number, pore size distribution, surface chemistry, and surface textural properties. The surface area and pore size distribution were measured by nitrogen adsorption method at â196 oC using a Surface Area Analyzer (Model: AUTOSORB â 1) in order to elucidate the microporosity of activated carbons. For nitrogen adsorption studies, the samples were degassed for 24 h at 50 oC to ensure complete evacuation of micropore. The iodine number was determined based on the Standard Test Method (ASTM D4607-94). In order to know the surface functional groups, the FT-IR spectra of ACC and ACS were recorded between 4000 and 450 cm-1 using a Perkin-Elmer Spectrum GX FT-IR/Raman spectrometer. Fifty scans were recorded at 4 cm-1 spectral resolution. For this study, the samples were ground and diluted to 0.5 wt% with spectroscopic grade KBr. A thin film was prepared from the resulting mixture using Perkin-Elmer manual hydraulic press. A scanning electron microscope (SEM) (Model JEOL 840) equipped with an energy dispersive X-ray microanalysis was used to determine the surface textural characteristics of the samples.
2.4Dye adsorption
Activated carbons, ACC and ACS were used for Methylene Blue dye adsorption from water. In each experiment 0.1 g of adsorbent was added into 50 mL dye solution in a conical flask. The flask was sealed with paraffin tape to avoid the evaporation and shaken for a desired length of time in a thermostatic orbital shaker at 25 oC. After adsorption, the solution was filtered out and the concentration of the residual dye solution was measured using a Visible Spectrophotometer (ANA â 75) at (max 626 nm. The same procedure was followed for a blank experiment to avoid any experimental error. The equilibrium adsorption of dye from different concentrations on ACC was measured to evaluate the Langmuir adsorption isotherm model. The amount of dye adsorbed, x/m was calculated and fitted to the following Langmuir Equation (3):
[pic] âŠâŠâŠâŠâŠâŠ(3)
where Ce is the dye concentration in the solution under equilibrium condition (mg/L), x/m is the total quantity of dye adsorbed per unit weight of ACC at equilibrium (mg/g), xm is the maximum monolayer adsorption capacity (mg/g), and KL is the Langmuir adsorption constant (L/mg) and it is related to the free energy of adsorption. The straight line was fitted to the points by the least square method, where the slope of the regression line is 1/xm and the intercept is 1/KL.1/ xm
3. Results and discussion
3.1. Yield, surface area and iodine number of activated carbon The activation burn-off, yield, specific surface area and iodine number of ACC and ACS are tabulated in Table 1. The mass decreased during activation is called activation burn-off. The yield was accounted by subtracting the activation burn-off from the total mass of biomass and it was 46.0% for ACC, while it is 12.0% for ACS. In the chemical activation, the bond cleavage initiated by the reaction of activating agent, ZnCl2 in jute stick resulted in the evolution of small molecules such as H2O, CH3OH, HCHO, CH3COOH, CO2 etc. These small gaseous products released from the carbon matrix, leaving behind the skeletal structure of carbons. This reaction took place on the outer surface as well as in the micro-pore surface, so as to increase the number of pores as well as the size of pores. The total amount of devolatilized products could be accounted to around 50.0% under optimum condition, which after washing and drying resulted in 46.0%.
The reactions occurred in the chemical activation process were influenced by activation temperature, and thus progressively increased the activation burn-off, resulted in reduced yield of ACC as we have observed in our previous work [22]. However, the BET surface area, which was created by the activation burn-off, increased only up to 500 oC. Further increase of temperature from 500 to 600 oC resulted in reduced surface area. This was due to the loss of some pore walls due to excessive carbon burn-off. For physical activation, the major part (around 70%) of the total burn-off occurred at the pyrolysis temperature at round 500 oC, while another 18% mass reduction occurred at activation temperature (700 oC). This huge burn-off resulted in very low yield (12%) of ACS as well as it was an indicative of less pore density but bigger pore size, and thus the surface area was also very low (730 m2/g) [22]. A further note revealed that the iodine number was much higher for ACC (2105 mg/g) than that of ACS (815 mg/g). It reflected to the predominant micropore structure of ACC, while it was macropore structure of ACS and it could be supported by the consistent reported results [28].
3.2. Pore size distribution
The pore size distribution has been analyzed by different methodes like Barrett, Joyner & Halenda (BJH), Dubinin-Astakhov (DA) and Density Functional Theory (DFT)/Monte-Carlo pore volume distribution using N2 adsorption isotherm under liquid nitrogen condition. Figure 1 illustrates the BJH cumulative adsorption pore volume distribution in terms of pore diameter. BJH adsorption was counted above the relative pressure 0.3 at which the spontaneous condensation of gas molecule in the cylindrical pores was predicted. As Figure 1, around 85% of total pore volume (1.21 mL/g) belongs to micropore (pore size 50 Ă ), respectively for ACC. However, around 48% of total pore volume (0.58 mL/g) belongs to micropore; whereas 21% and 31% of total pore volume belong to mesopore and macropore size, respectively for ACS. The DA method is often used to evaluate the micropore of activated carbons. In the DA equation, there are two variable parameters, (Eo) and n. Eo denoted as average adsorption energy, related to the pore diameter and n denoted the width of the energy distribution, which was related to the pore size distribution.
The values of n higher than 2 represents the homogeneous micropore structure of activated carbon, while lower than this value represents the heterogeneity of pores in meso- and macropore range. As in Figure 2, the maxima of the micropore volume was much higher for ACC than that of ACS. It implied that the majority of the pore volume of ACC was related to the micropore structure compared to that of the ACS. It could be more clearly elucidated by the DFT/Monte-Carlo pore size distribution histogram as illustrated in Figure 3. The figure illustrates that the sample ACC predominantly featured the micropore volume of 12-15 Ă pore width, while it was almost one fourth for ACS. The figure also represents that a minor fraction of pore volume was related to macropore region for ACC. Meanwhile, the pore volume of ACS is distributed in a wide range of macropore region. Therefore, it could be predicted that the micropore structure of ACC can adsorb methylene blue dye much more effectively than that of ACS, which is discussed in the subsequent sections.
3.3. Surface functionality
The existence of organic functional groups on the activated carbon surface provides surface polarity, which in turn contributes to adsorption properties. The number of exposed functional groups also depends on the surface area. Hence the wide surface area with randomly distributed organic functional groups on activated carbon could presumably adsorb dye molecule more effectively. The functional groups on activated carbon could be identified by using FT-IR spectroscopy. Figure 4 represents the FT-IR spectra of ACC and ACS over the wavelength range of 4000-450 cm-1. The broad band at 3210 cm-1 for ACC was assigned to OH- stretching vibration. It seems that the band shifted from its usual position at around 3400 cm-1 to lower wavenumber 3210 cm-1 and is too broad, implies that the âOH group in ACC was not completely free but more likely cross-linked. This was due to the fact that, ZnCl2 initiated bond cleavage resulted in dehydration, elimination and cross-linking reactions.
The band at 2900 cm-1 and 1740 cm-1 in raw jute stick was completely disappeared from the ACC, leading to the significant decrease of aliphaticity in the activated carbon [22]. The newly generated band at 1690 cm-1 was attributed to stretching vibration of aryl ketone, while the strong band at 1580 cm-1 ascribed to the C=O stretching of carbonyl group in quinone structure [23]. The cross linking and condensation of aromatic ring attributed to more condensed polyaromatic ring system, which was attributed to the bands at 1170 and 1070 cm-1 for C-C-C bending. In contrast, the bands with much lower intensity were appeared for ACS. This could be due to the release of IR sensitive functional groups and aliphatic groups from ACS because it was treated at high temperature (700oC).
4. Textural characterization by scanning electron microscopy (SEM)
Figure 5 illustrates the Scanning Electron Microscopic images of ACC and ACS. It clearly exhibits the morphological difference of two different activated carbons prepared by different activation methods. The chemical activation method generated a honeycomb type porous carbon skeleton, while steam activation created pores on the surface far apart from each other. It is obvious that the pore density of ACC is much higher than that of ACS. However, as Figure 5, the visible pore size is in micrometer range (macropore), 1000 times higher than that of the nanometres. Nevertheless, the wide surface area and micropore volume, as revealed from the nitrogen adsorption study, were assumed to be the contribution of micropore size. It seems that, although it is not visible under the magnification used a wide range of micropores are randomly distributed on the wall of the visible pores in ACC.
3. 5. Adsorption of Methylene Blue dye (MB)
Methylene Blue is one of the representative basic dyes widely used in textile, lather and paper industries. It is also used as a model adsorbate to determine the solution phase adsorption capacity of carbon based adsorbent. Figure 6 illustrates the MB adsorption isotherm of two different adsorbents as a function of adsorption time at 30 oC. The equilibrium adsorption not only depends on adsorption time but also depends on the initial concentration of dye solution. The objective of this experiment was to determine the maximum MB adsorption capacity of both activated carbons in a specific adsorption time. Hence, the initial concentration of dye was comparatively higher (1000 mg/L) than that of usual adsorption studies [29, 30]. As Figure 6, ACS has very limited adsorption capacity compared to the ACC. The equilibrium adsorption of MB on ACS obtained was 210 mg/g even after 80 h. The equilibrium adsorption was attained in 10 h for ACC with much higher adsorption capacity (470 mg/g) than that of ACS as well as many reported activated carbons [30]. From the characterization of ACC and ACS, it was revealed that two adsorbents have completely different structural features with different surface functionality, which could feature the different adsorption mechanism.
For ACC, most of the MB dye adsorbed on the micropore surface with stronger attraction force due to the presence of oxygen containing polar functional groups. It was also predicted that the micropores were completely filled with dye molecules irreversibly. Because of wide micropore surface of ACC with strong attraction force the equilibrium adsorption was much faster than ACS. In contrast, the adsorption on the macropore surface with less functional groups of ACS was resulted in weakly forced adsorption. The adsorbed MB molecules spontaneously desorbed, and thus it took longer time to attain equilibrium adsorption with much lower adsorption capacity compared to ACC. Figure 7 illustrates the effect of temperature on adsorption of MB using ACC and ACS. Temperature can affect adsorption in two competitive ways. Firstly, it can improve the diffusion rate of adsorbate molecules across the external boundary layer and internal pores of the adsorbent, so as to increase the adsorption rate and secondly, it can weaken the force between adsorbate and adsorbent, so as to enhance the desorption rate. In MB dye adsorption by ACC, the amount of adsorption significantly increased with increasing temperature up to around 60 oC, while it was constant up to 80 oC.
Further increase of temperature resulted in decreasing trend of adsorption. It could be predicted that the diffusion rate predominantly promoted the adsorption rate up to 60 oC and that was almost equal to desorption rate in between 60 and 80 oC. Above 80 oC, the desorption rate became prominent, and thus the adsorption decreased. The adsorption of MB molecules on ACS slightly increased with temperature up to 70 oC. This could be due to the less diffusion resistance for MB molecule to travel through macropore channel of ACS. The pH is one of the most important factors that control the adsorption of dye onto adsorbent. As IR studies, activated carbon surface possesses some oxygen containing functional groups. Those functional groups as well as the carbon in the aromatic ring system of activated carbon would be influenced by H+ and OH- ions at low and high pH, respectively. At low pH, where the H+ ion was dominant in the solution, the species on the surface was assumed to be protonated, and thus the surface positive charge assumed to be increased.
During adsorption of MB, a positively charged dye, the surface charge partially repulsed the dye molecules; hence the dye uptake was lower at lower pH for both ACC and ACS as shown in Figure 8. As pH increased, the dye uptake increased significantly up to pH 7. For ACC the isotherm remained plateau in between pH 7 to pH 11; however, dye uptake jumped from 300 mg/g to 370 mg/g when pH changed from 11 to 13. It could be predicted that in the range of pH 7-11, the surface charge remained at zero point charge (pHZPC), where the adsorbent and adsorbate could utilize their inherent nature in adsorption. However, when OH- ion became dominant in the solution at higher pH (>pHZPC), the adsorbent surface preferentially converted to negatively charge. Hence, the electrostatic attraction between positively charged dye molecules and negatively charged activated carbon surface significantly increased, which attributed to the sudden increase of dye adsorption by ACC.
3.6. Adsorption isotherm
Adsorption of solute from solution influenced by a number of parameters as discussed in the previous sections. Under the suitable conditions, the maximum monolayer adsorption capacity is an important indicator of the quality of an adsorbent. This could be predicted by Langmuir adsorption isotherm, which is a most frequently used equation to characterize adsorbents, especially activated carbons. The Equation 3 is a linear regression of the Langmuir equation used for MB dye adsorption using ACC. The maximum monolayer adsorption capacity was calculated to be 303 mg/g from the slope of the linear regression line. The correlation coefficient of the linear regression line obtained was R2=0.991, which was highly significant to fit the data well to the Langmuir equation. The dimensionless separation factor of the equilibrium parameter, RL is the essential feature of the Langmuir adsorption isotherm, which defines the favourable nature of adsorption. This can be calculated by the following Equation 4: [pic]âŠâŠ.(4) where C0 is the highest initial dye concentration (mg/L) of the solution. The favourable value of RL lies between 0 and 1. The experimental value of RL obtained was 0.0002, leading to the favourable adsorption of MB onto the ACC [29, 30].
4. Conclusion
The nitrogen adsorption studies revealed that the micropore structure with wide surface area was developed in chemically activated carbon (ACC), while macropore structure with less surface area was developed in physically activated carbon (ACS). In addition the surface functional groups of ACC are more significant than that of ACS. The micropore structure with organic functional groups on the surface favoured dye adsorption more efficiently for ACC. At equilibrium, around 480 mg/g MB dye was adsorbed on ACC, while it was much lower for ACS (182 mg/g). The data of Methylene Blue dye adsorption from different initial concentrations of solution was quite fitted to the Langmuir adsorption model, which provided the maximum monolayer adsorption capacity, 303 mg/g. The separation factor RL was obtained to be 0.002 lies between 0 and 1, which was favourable value for ACC for efficient adsorption of Methylene Blue.
Acknowledgement
This research was financially supported by the Third World Academy of Sciences (TWAS) under the project no.: 07-033 LDC/CHE/ASâUNESCO FR: 3240144818 and The Ministry of Science and Information and Communiction Technology (MOSICT 013A/06). The authors are grateful to the Faculty of Chemical Engineering, Universiti Teknologi Mara for providing the laboratory facilities to conduct a major part of this work.
References
[1]Crini G. Non-conventional low-cost adsorbents for dye removal: A review. Bioresour Technol 2006;97(9):1061-85. [2]Crini G, Gimbert F, Robert C, Martel B, Adam O, Morin-Crini N, Giorgi FD, Badot PâM. The removal of Basic Blue 3 from aqueous solutions by chitosan-based adsorbent: Batch studies. J Hazard Mater 2008;153(1-2):96â106. [3]Crini G, Peindy HN, Gimbert F, Robert C. Removal of C.I. Basic Green 4 (Malachite Green) from aqueous solutions by adsorption using cyclodextrin-based adsorbent: Kinetic and equilibrium studies. Sep Purif Technol 2007;53(1):97â110. [4]Crini G, Peindy HN. Adsorption of C.I. Basic Blue 9 on cyclodextrin-based material containing carboxylic groups. Dyes Pigments 2006;70(3):204-11. [5] Elahmadi MF, Bensalah N, Gadri A. Treatment of aqueous wastes contaminated with Congo Red dye by electrochemical oxidation and ozonation processes. J Hazard Mater 2009;168(2â3):1163-9. [pic]Show preview [6]Soon AN, Hameed BH. Heterogeneous catalytic treatment of synthetic dyes in aqueous media using Fenton and photo-assisted Fenton process. Desalination 2011; 269(1â3):1-16. [7]TĂŒrgay O, Ersöz G, Atalay S, Forss J, Welander U. The treatment of azo
dyes found in textile industry wastewater by anaerobic biological method and chemical oxidation. Sep Purif Technol 2011;79(1):26-33. [8]Guibal E, Roussy J. Coagulation and flocculation of dye-containing solutions using a biopolymer (Chitosan). React Funct Poly 2007;67(1):33-42. [9]He Y, Li G, Wang H, Zhao J, Su H, Huang Q. Effect of operating conditions on separation performance of reactive dye solution with membrane process. J Memb Sci 2008;321(2):183â9. [10]Lata H, Gupta RK, Garg VK. Removal of basic dye from aqueous solution using chemically modified Parthenium Hysterophorus Linn biomass. Chem Eng Commun 2008;195(10):1185-99. [11]Lata H, Mor S, Garg VK, Gupta RK. Removal of a dye from simulated waste water by adsorption using treated parthenium biomass. J Hazard Mater 2008;153(1-2):213-20. [12]Wang DW, Li F, Lu GQ, Cheng HM. Synthesis and dye separation performance of ferromagnetic hierarchical porous carbon. Carbon 2008;46(12):1593-9. [13]Dias JM, Alvim-Ferraz MCM, Almeida MF, Rivera-Utrilla J, Sanchez-Polo M. Waste materials for activated carbon preparation and its use in aqueous-phase treatment: A review J Env Manag 2007;85:833-46. [14]Seredych M, Deliyanni E, Bandosz TJ. Role of microporosity and surface chemistry in adsorption of 4,6-dimethyldibenzothiophene on polymer-derived activated carbons. Fuel 2010;89( 7):1499-07. [15]Silvestre-Albero A, Silvestre-Albero J, SepĂșlveda-Escribano A, RodrĂguez-Reinoso F. Ethanol removal using activated carbon: Effect of porous structure and surface chemistry. Microporous Mesoporous Mater2009;120(1â2):62-8. [16]Sing KSW, Everett DH, Haul RAW, Moscou L, Pierotti RA, Rouquerol J, Siemieniewska T. Reporting physisorption data for gas/solid systems. Pure Appl Chem 1985;57(4):603-19. [17]Zhao W, Fierro V, Zlotea C, Aylon E, Izquierdo MT, Latroche M, Celzard A. Activated carbons with appropriate micropore size distribution for hydrogen adsorption. Int J Hydrogen Energ, 2011;36(9):5431-4. [18]Mui ZLK, Cheung WH, Valix M, McKay G. Dye adsorption onto activated carbons from tyre rubber waste using surface coverage analysis. J Colloid Interface Sci 2010;347(2):290-300. [19]Skodras G, Diamantopoulou I, Zabaniotou A, Stavropoulos G, Sakellaropoulos GP. Enhanced mercury adsorption in activated carbons from biomass materials and waste tires. Fuel Proc Technol 2007;88:749-58. [20]Olivares-MarĂn M, FernĂĄndez-GonzĂĄlez C, MacĂas-GarcĂa A, GĂłmez-Serrano V. Preparation of activated carbon from cherry stones by physical activation in air. Influence of the chemical carbonization with
H2SO4. J Anal Appl Pyrol 2012;94:131â7. [21]Kalderis D, Koutoulakis D, Paraskeva P, Diamadopoulos E, Otal E, del Valle JO, Fernandez-Pereira C. Adsorption of polluting substances on activated carbons prepared from rice husk and sugarcane bagasse. Chem Eng J 2008;144(1):42-50. [22]Asadullah M, Asaduzzaman M, Kabir MS, Mostofa MG, Miyazawa T. Chemical and structural evaluation of activated carbon prepared from jute sticks for Brilliant Green dye removal from aqueous solution, J. Hazard. Mater. 2010;174:437â43. [23]Boehm HP. Surface oxides on carbon and their analysis: a critical assessment. Carbon 2002;40:145-9. [24]Keown DM, Hayashi J-I, Li C-Z. Drastic changes in biomass char structure and reactivity upon contact with steam. Fuel 2008;87:1127-32. [25]Asadullah M, Miyazawa T, Ito S-I, Kunimori K, Yamada M, Tomishige K. Gasification of different biomasses in a dual-bed gasifier system combined with novel catalysts with high energy efficiency. Appl Catal A: General 2004;267(1-2):95-102. [26]Asadullah M, Rahman MA, Motin MA, Sultan MB. Preparation and adsorption studies of high specific surface area activated carbon obtained from chemical activation of jute stick. Ads Sci Technol 2006;24(9):761-70. [27]Asadullah M, Rahman MA, Motin MA, Sultan MB. Adsorption studies of activated carbon derived from steam activation of jute stick char. J Surf Sci Technol 2007;23(1-2):1-8. [28]Cuerda-Correa EM, MacĂas-GarcĂa A, DĂaz DĂez MA, Ortiz AL. Textural and morphological study of activated carbon fibers prepared from kenaf. Micropore Mesopore Mater 2008;111(1-3):523-9. [29]Ahmad AL, Loh MM, Aziz JA. Preparation and characterization of activated carbon from oil palm wood and its evaluation on Methylene Blue adsorption. Dyes Pigments 2007;75:263-72. [30]Gerçel Ă, Ăzcan A, Ăzcan AS, Gerçel HF. Preparation of activated carbon from a renewable bio-plant of Euphorbia rigida by H2SO4 activation and its adsorption behavior in aqueous solutions. Appl Surf Sci 2007;253(11):4843-52.
Figure captions
Fig. 1. Barrett, Joyner & Halenda (BJH) cumulative adsorption pore volume, (a) and cumulative adsorption surface area, (b). Fig. 2. Pore size distribution measured by Dubinin-Astakhov method for ACC and ACS. Fig. 3. Density Functional Theory (DFT)/Monte-Carlo pore volume histogram of ACC and
ACS. Fig. 4. FT-IR spectra of ACC and ACS.
Fig. 5. Scanning electron micrograph of ACC and ACS.
Fig. 6. Effect of contact time on Methylene Blue dye adsorption on ACC and ACS at 25 oC. Fig. 7. Effect of temperature on Methylene Blue dye adsorption on ACC and ACS. Fig. 8. Effect of pH on Methylene Blue dye adsorption at 30 oC on ACC and ACS.