Biomass Energy and Biofuels
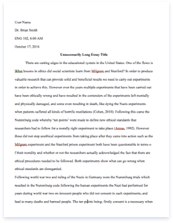
- Pages: 9
- Word count: 2165
- Category: Carbon
A limited time offer! Get a custom sample essay written according to your requirements urgent 3h delivery guaranteed
Order NowThanks to the industrial revolution that made it possible for exponential economic growth, human beings are currently living in an era characterized by the staggering amount of annual energy consumption. As we become extremely dependent on finite and diminishing energy sources such as coal, oil and gas, the Earth suffers from overexploitation and is at stake. The top priority of human beings thus becomes to transition to the use of renewable energy. Among the possible sources of renewable energy, biomass accounts for the largest proportion. Biomass is the biodegradable part of waste and remains resulting from agricultural, forestrial and related productions (de Vos, 2006). It is a major source of carbon that can renew itself over a short time span in order to maintain and supplement energy supplies (Klass, 1998). Biomass is recognized by many governments and policy makers as a feasible domestic energy resource that has the potential of reducing oil consumption and mitigating the dependency on imported oil (Klass, 1998).
Historically, biomass has been one of the oldest forms of energy. Along with other renewables sources such as hydro, wind and solar energy, it was the dominant energy source globally, until it was replaced by coal in the beginning of the 18th century due to the increasing scarcity of wood fuel (Radetzki, 1997). Solar energy plays an indispensable role in the growth of biomass, as it is captured as fixed carbon in biomass through photosynthesis, during which carbon dioxide is converted to organic compounds (Klass, 1998). Normally, biomass is gathered to provide forage, food, fiber, and materials of construction or is left in the growth areas where the naturally decomposing biomass theoretically can be partially recovered as fossil fuels after a long period of time; alternatively, biomass and its processing waste could also be converted directly into synthetic organic fuels if suitable conversion processes were available (Klass, 1998). With efforts being put into producing and efficiently using biomass, it can be a universally available and flexible fuel source with most of the world’s population residing in developing countries that usually lack fossil fuels and means to import them.
There are several ways to convert biomass into energy. The most conventional and common way is through direct combustion, which is used to generate electricity. However, the efficiency of such way of electricity generation is low (Küçük & Demirbaş, 1997). Besides being used as a solid fuel, biomass can also be converted into liquid or gas to produce electricity, heat, chemicals, or fuels in gaseous and liquid forms (Demirbas, 2009). This process is known as thermochemical conversion, and it can be divided into three categories: pyrolysis, gasification, and liquefaction (Demirbas, 2009). Pyrolysis occurs when organic matter thermally decomposes without oxygen being present or when considerably less oxygen is present than required for complete combustion (Demirbas, 2009). The fuel produced through pyrolysis is liquid pyrolysis oil, sometimes called bio-oil, which can be burned like fuel oil or refined into chemicals and fuels. Gasification is a process that involves mixing biomass with air, oxygen, or steam to convert it into gaseous products such as hydrogen, methane, and carbon dioxide (Swain, Das, & Naik, 2011).
It can add value to low value materials by converting them to profitable fuels and products. Liquefaction consists of the thermal decomposition of feedstock large molecules into fragments of light molecules with a suitable catalyst being present, and then the unstable fragments can polymerize again into oily compounds (Alonso, Bond, & Dumesic, 2010). Another way to convert biomass is through biochemical conversion, the process by which biomass is broken down into gas, waste, and water by using enzymes and other microorganisms (Küçük & Demirbaş, 1997). The biochemical processes refer mainly to aerobic fermentation that produces compost, carbon dioxide and water, anaerobic fermentation that produces fertilizer, and gas and alcoholic fermentation that produces ethanol (Küçük & Demirbaş, 1997). One advantage of anaerobic fermentation is that it solves the pollution problem while also producing energy and organic fertilizer from a renewable source (Küçük & Demirbaş, 1997). Biochemical conversion process is attractive because the start-up and maintenance costs are significantly lower than for thermochemical plants (Gomez, Steele-King, & McQueen-Mason, 2008).
Biochemical systems are also considered one of the most promising and environmentally sustainable alternatives for reducing carbon dioxide levels in the atmosphere. Unlike the burning of fossil fuels, the combustion of biofuels has the potential to be carbon neutral, due to the fact that, principally, biofuels have lower carbon emissions as they are produced within the short-term carbon cycle, and the amount of CO2 they release to the atmosphere through combustion is only as much as the plant growth has taken out (Gomez et al., 2008). Therefore, the carbon dioxide produced during fuel combustion can be consumed by subsequent biomass regrowth. Currently, crop-based bioethanol and biodiesel are the most widely used biomass-derived fuels, and they have been successfully implemented in the transportation sector as alternatives to petrol-based gasoline and diesel (Gomez et al., 2008).
Bioethanol and biodiesel, referred to as first-generation biofuels, are both produced from commodities that are also used for food; the difference is that biodiesel is made from the same oil crops used in the food industry, and bioethanol is produced by the fermentation of sugars or derived from the hydrolysis of starch (Gomez et al., 2008). Second-generation biofuels are produced from non-food biomass and thus they can be more sustainable. They are largely lingo-cellulosic materials including by-products such as forest residues, wastes and dedicated feedstocks (Sims, Mabee, Saddler, & Taylor, 2009). At present, the production of such fuels is not cost-effective because there are a number of technical barriers that need to be overcome before their potential can be realized (Gomez et al., 2008). However, once second-generation biofuel technologies are fully commercialized, it is likely they will be favored over many first-generation alternatives with objectives such as environmental performance or security of supply (Sims, Mabee, Saddler, & Taylor, 2009). Because plant biomass represents one of the most abundant and biological resources on Earth, it can be a promising source of material for fuels and raw materials. Among the biofuels, ethanol is the one that has received wide attention, and it has already been produced in large scale, including Brazil and the United States (Macedo, Seabra, & Silva, 2008).
The starting point in the ethanol production process is to provide the yeast with a source of carbohydrates such as corn and sugarcane, and allow it to use the carbohydrates in fermentation, during which the carbohydrates are broken down by the to release energy for the use of metabolic processes (Banschbach & Letovsky, 2010). The fermentation must be followed by distillation to remove excess water in the mixture to reach the desired concentration (Banschbach & Letovsky, 2010). Brazil is the world leader in producing sugarcane-based ethanol, and through investing in this process it has successfully achieved independence from oil imports (Banschbach & Letovsky, 2010). Furthermore, in Brazil, the land devoted to sugarcane are much more effective in ethanol production than land devoted to corn, and also Brazilian ethanol refineries are able to cut greenhouse gas emissions through deriving most of their energy from burning sugarcane residue (Banschbach & Letovsky, 2010). However, one problem with sugarcane-based ethanol is that it may not be produced year-round, since ethanol can be produced during harvest season and sugarcane might lose juice if stored too long. Another serious problem with using sugarcane for ethanol production is that it can contribute to the deforestation of the rainforests in Brazil.
If the rainforests are eliminated to make room for sugarcane production, the carbon dioxide previously stored in the forests will be released into the atmosphere through cutting and burning of trees and decay of roots (Banschbach & Letovsky, 2010). In the U.S., corn is the main feedstock ingredient used to produce ethanol. Corn-based ethanol is already widely used in the United States, but it is blended into conventional gasoline rather than used as a stand-alone fuel for cars because it promotes more complete combustion and therefore reduce smog (Banschbach & Letovsky, 2010). However, critics claim that both sugarcane- and corn-based production of ethanol can potentially drive food prices up and decrease global food security (Banschbach & Letovsky, 2010). If the demand for corn soars in order to satisfy the rising number of ethanol refineries, corn prices are also likely to rise, which might result in higher prices for consumers on a wide range of food products. Production of corn-based ethanol can also be water-intensive (Banschbach & Letovsky, 2010).
The amount of water used in the refining process is equivalent to the water demand of a small town (Banschbach & Letovsky, 2010). Moreover, corn is one of the most intensive crops that deplete nutrients and important minerals from the soil. If the corn stover is used in production of ethanol, the minerals and nutrients are removed from the soil and the soil is not replenished (Banschbach & Letovsky, 2010). Because of these drawbacks, other forms of biomass are being explored and considered as alternatives to corn-based ethanol. One alternatives to corn ethanol is cellulosic ethanol. Cellulosic ethanol is an alternative fuel that is derived from cellulose instead of starch, and it is considered as a second-generation biofuel. Because second-generation biofuels utilize a wide range of materials in fuel production such as wastes, cellulosic ethanol can be produced almost anywhere. In addition, cellulosic ethanol may offer better engine performance (Somma, Lobkowicz, & Deason, 2010). Due to the fact that cellulosic feedstocks have higher energy content compared to corn feedstocks, it requires less cropland to produce cellulosic ethanol than to produce corn for equivalent amounts of energy (Somma et al., 2010).
However, at present no cellulosic ethanol is on the market, mainly due to the fact that cellulosic production costs are significantly higher than that of corn ethanol and other alternative fuels because existing refining processes are very expensive and involve many steps (Somma et al., 2010). As a renewable resource, biofuel offers a lot of benefits over traditional fossil fuels, and it has the potential to provide a cleaner and more sustainable source of energy for the transportation sector. Second-generation biofuels are considered to be more sustainable than first-generation fuels as they rely on crop and forest residues. Because the immediate use of first-generation biofuels requires changes such as modification of engines and production plants which cannot be accomplished in a short period of time, the transition to the second generation of biofuels seems to be more economically convenient (Gomez et al., 2008).
If the biofuel industry is well established, it has the potential to provide significant environmental as well as economic benefits, such as the reduction of the dependence on oil imports and price fluctuations new job creation in multiple sectors, and development of rural areas to better utilize the croplands. However, controversies arise with respect to the “food or fuel” dilemma. Setting aside lands to grow biomass feedstocks for biofuels production leads to land competition with food production. This controversy might be solved if relevant researches and development are implemented that focus on the utilization of more abundant and plant biomass, allowing sustainable production of biofuels without affecting food supplies or forcing changes in land use. In brief, biofuels cannot completely replace coal in the short term. In order to solve the present energy crisis, multiple efforts and investments will have to be made besides the use of biofuels, including solar, wind, and various other sources of non-coal-based fuels.
References
Alonso, D.M., Bond, J. Q., & Dumesic, J. A. (2010). Catalytic conversion of biomass to biofuels. Green Chemistry, 12, 1493-1513. doi: 10.1039/c004654j Banschbach, V. S., & Letovsky, R. (2010). The Use of Corn Versus Sugarcane to Produce Ethanol Fuel: A Fermentation Experiment for Environmental Studies. The American Biology Teacher January, 72(1), 31-36. doi: 10.1525/abt.2010.72.1.8 Demirbas, A. (2009). Biofuels securing the planet’s future energy needs. Energy Conversion and Management, 50(9), 2239-2249. doi:10.1016/j.enconman.2009.05.010 de Vos, R. (2006). Defining biomass. Refocus, 7(5), 58-59. Retrieved from http://journals.ohiolink.edu/ejc/pdf.cgi/de_Vos_Rolf.pdf?issn=14710846&issue=v07i0005&article=58_db Gomez, L. D., Steele-King, C. G., & McQueen-Mason, S. J. (2008). Sustainable Liquid Biofuels from Biomass: The Writing’s on the Walls. New Phytologist, 178(3), 473-485. Retrieved from http://www.jstor.org.proxy.lib.ohio-state.edu/stable/pdfplus/30142310.pdf?acceptTC=true Klass, D. L. (1998). Biomass as an Energy Resource: Concept and Markets. In Biomass for Renewable Energy, Fuels, and Chemicals. Retrieved from http://www.knovel.com.proxy.lib.ohio-state.edu/web/portal/browse/display?_EXT_KNOVEL_DISPLAY_bookid=2245 Küçük, M.M., & Demirbaş, A. (1997). Biomass conversion processes. Energy Conversion and Management, 38(2), 151–165. http://dx.doi.org/10.1016/0196-8904(96)00031-3 Macedo, I. C., Seabra, J. E.A., & Silva, J. E.A.R. (2008). Green house gases emissions in the production and use of ethanol from sugarcane in Brazil: The 2005/2006 averages and a prediction for 2020. Biomass and Bioenergy, 32, 582-595. doi:10.1016/j.biombioe.2007.12.006 Radetzki, M. (1997). The economics of biomass in industrialized countries: an overview. Energy Policy, 25(6), 545–554. http://dx.doi.org/10.1016/S0301-4215(97)00043-8 Sims, R.E.H, Mabee, W., Saddler, J.N., & Taylor, M. (2009). An overview of second generation biofuel technologies. Bioresource Technology, 101, 1570–1580. doi:10.1016/j.biortech.2009.11.046 Somma, D., Lobkowicz, H., & Deason J. P. (2010). Growing America’s fuel: an analysis of corn and cellulosic