Earthquake resistant design
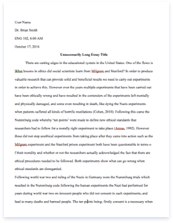
- Pages: 13
- Word count: 3132
- Category: Earthquakes
A limited time offer! Get a custom sample essay written according to your requirements urgent 3h delivery guaranteed
Order NowEARTHQUAKE
An earthquake (also known as a tremor, temblor or seismic activity) is the result of a sudden release of energy in the earthâs crust that creates SEISMIC WAVES. Earthquakes are measured with a seismometer; a device which also records is known as a seismograph.
SEISMIC DEFORMATION
When an earthquake fault ruptures, it causes two types of deformation: static; and dynamic. Static deformation is the permanent displacement of the ground due to the event. The earthquake cycle progresses from a fault that is not under stress, to a stressed fault as the plate tectonic motions driving the fault slowly proceed, to rupture during an earthquake and a newly-relaxed but deformed state.
Typically, someone will build a straight reference line such as a road, railroad, pole line, or fence line across the fault while it is in the pre-rupture stressed state. After the earthquake, the formerly stright line is distorted into a shape having increasing displacement near the fault, a process known as elastic rebound.
SEISMIC WAVES
The second type of deformation, dynamic motions, is essentially sound waves radiated from the earthquake as it ruptures. While most of the plate-tectonic energy driving fault ruptures is taken up by static deformation, up to 10% may dissipate immediately in the form of seismic waves. The mechanical properties of the rocks that seismic waves travel through quickly organize the waves into two types. Compressional waves, also known as primary or P waves, travel fastest, at speeds between 1.5 and 8 kilometers per second in the Earth’s crust.
Shear waves, also known as secondary or S waves, travel more slowly, usually at 60% to 70% of the speed of P waves. P waves shake the ground in the direction they are propagating, while S waves shake perpendicularly or transverse to the direction of propagation. Although wave speeds vary by a factor of ten or more in the Earth, the ratio between the average speeds of a P wave and of its following S wave is quite constant. This fact enables seismologists to simply time the delay between the arrival of the P wave and the arrival of the S wave to get a quick and reasonably accurate estimate of the distance of the earthquake from the observation station. Just multiply the S-minus-P (S-P) time, in seconds, by the factor 8 km/s to get the approximate distance in kilometers.
The dynamic, transient seismic waves from any substantial earthquake will propagate all around and entirely through the Earth. Given a sensitive enough detector, it is possible to record the seismic waves from even minor events occurring anywhere in the world at any other location on the globe. Nuclear test-ban treaties in effect today rely on our ability to detect a nuclear explosion anywhere equivalent to an earthquake as small as Richter Magnitude 3.5. SEISMOGRAPHS AND SEISMOGRAMS
EARTHQUAKES AFFECT ON NATURAL ENVIRONMENT
Liquefaction
Strong ground motion during an earthquake can cause water-saturated, unconsolidated soil to act more like a dense fluid than a solid; this process is called liquefaction. Landslides
Tsunamis and Seiche
Tsunami (âSoo na meâ) is Japanese for tidal wave. Faults
EARTHQUAKE AND CHARACTERISTIC OF BUILDINGS
Several important characteristics of buildings affect performance during an earthquake. Buildings of different construction materials or configurations will respond in different ways to the same ground motion; some may collapse while others survive Natural Period.
All objects (including buildings and the ground) have a ânatural period,â or the time it takes to swing back and forth, from point A to point B and back again. As seismic waves move through the ground, the ground also moves at its natural period. This can become a problem if the period of the ground is the same as that of a building on the ground. When a building and the ground sway or vibrate at the same rate, they are said to resonate. When a building and the ground resonate it can mean disaster. This is because, as the building and ground resonate, their vibrations are amplified or increased, and greater stress is placed on the building.
One of the most important factors affecting the period is height. A taller building will swing back and forth more slowly (or for a longer period) than a shorter one. For example, a 4-story building might have a natural period of 0.5 seconds, while a 60-story building may have a period of as much as 7 seconds. Building height can have dramatic effects on a structureâs performance in an earthquake. A taller building often suffers more damage than a shorter one because the natural period of the ground tends to match that of buildings nine stories or taller. This explains why some buildings are severely damaged and others are not. Damping
One way an architect or engineer may decrease the effects of resonance is by constructing buildings so that the vibration of a building is quickly reduced as an earthquake sets it in motion. This is called damping, the termination or retardation of the motion or vibration of a structure. Connections of nonstructural elements such as partitions, ceilings, and exterior walls can dampen a buildingâs vibration. Modern office buildings with open flooring and few partitions tend to be deficient in damping and therefore suffer more damage in an earthquake. It is most advantageous for a building to have a high level of damping characteristicsâin effect to be an inefficient vibrator. With damping design, a building is less likely to resonate in tune with the ground. Ductility and Strength
Ductility is another factor that can affect the performance of a building during an earthquake. Ductility is the property of certain materials to fail only after large stresses and strains have occurred. Figure 4-8 illustrates what we mean by ductility. Brittle materials, such as non-reinforced concrete, fail suddenly with minimum tensile stresses, so plain concrete beams are no longer used. Other materials, primarily steel, bend or deform before they fail. We can rely on ductile materials to absorb energy and prevent collapse when earthquake forces overwhelm a building. In fact, adding steel rods to concrete can reinforce it and give the concrete considerable ductility and strength. Concrete reinforced with steel will help prevent it from failing during an earthquake.
Stiffness
A building is made up of both rigid and flexible elements. For example, beams and columns may be more flexible than stiff concrete walls or panels. Less rigid building elements have a greater capacity to absorb several cycles of ground motion before failure, in contrast to stiff elements, which may fail abruptly and shatter suddenly during an earthquake. Earthquake forces automatically focus on the stiffer, rigid elements of a building. For this reason, buildings must be constructed of parts that have the same level of flexibility, so that one element does not bend too much and transfer the energy of the earthquake to less ductile elements of the building.
Drift
Drift is the extent to which a building bends or sways. Limits are often imposed on drift so a building is not designed to be so flexible that the resulting drift or swaying during an earthquake causes excessive damage. Figure 4-10 shows how a building can be affected by drift in an earthquake. If the level of drift is too high, a building may pound into the one next to it. Or the building may be structurally safe but nonstructural components, such as ceilings and walls, could be damaged as the building bends and the ceilings and walls are ripped away from their attachments. Of course, people in the building could be killed or injured from falling debris.
Building Configuration
Configuration of a building determines the ways in which seismic forces are distributed throughout the building. Earthquakes have earned a reputation for their ability to find and exploit the weak link in buildings. Generally speaking, a building with a symmetrical design and balanced resistance will hold up best. Letâs look at several types of buildings that illustrate this point.
L-shaped Buildings
During an earthquake, an L-shaped building will experience increased stress at the point where the wings of the building meet. The difference in stiffness between the two wings causes this stress. To illustrate this point, letâs think for a moment about the two wings of our L-shaped building as separate buildings.
Letâs say the force of an earthquake travels parallel to building A. The orientation of building A to the earthquake results in it swaying less than building B. This means that the perpendicular building, building B, will sway more than the parallel building, building A. Now letâs put the buildings back together into an L shape, as shown in Figure
In an L-shaped building, the two wings are forced to move together. As the two wings pull and push on each other, high stresses occur at the point where the two wings are joined. This problem also occurs in buildings with a T, H, or + shape. Torsional forces also affect the way an L-shaped building reacts during an earthquake. Torsional forces are forces that make an object rotate. They are created in a building by a lack of balance in forces. These forces build up as the B wing (perpendicular) attempts to rotate around the stiffer A wing (parallel). Another way to illustrate torsional forces is to think about moving a piano. To move the piano you would be careful to push it from the center. If you pushed at only one end, the piano would rotate around its center of mass, as shown in Figure below. Torsional forces create this rotating effect. The same thing happens to L-, T-, H-, or +-shaped buildings. Torsional forces on these buildings can cause one wing to rotate around the other. If buildings are not designed to resist torsional forces, considerable damage or collapse could occur.
SOFT-STORY
A soft-story building is a structure with stiffer, more rigid upper stories and an open, flexible first story. This design is found in buildings where the first story contains a parking garage or an open commercial area for stores and the upper floors house offices or apartments. This design creates a discontinuity of strength and stiffness. If all stories are approximately equal in strength, the entire building would bend in an earthquake. If the first story is softer, or more flexible, than the other stories, the bending would concentrate there. Because the first floor is also the most highly loaded, the problem is compounded, thus possibly causing column failure .This also will put additional stress on the connection between the first and second stories and can cause the building to collapse. COMPONENTS OF BUILDINGS THAT RESIST EARTHQUAKE
Diaphragms
The floor and roof systems that distribute an earthquakeâs lateral forces are referred to as diaphragms. Diaphragms support the gravitational and lateral forces on a building and transfer them to vertical structural elements like shear walls, braced frames, and moment-resistant frames. These vertical elements help resist lateral forces and are therefore called horizontal (or lateral) bracing systems.
Horizontal Bracing Systems
Three horizontal bracing systems can be used to resist earthquake forces. These are: âą Shear wall systems,
ï
âą Braced frame systems, and
âą Moment-resistant systems.
Shear Wall System
Walls within a building that are designed to receive horizontal forces parallel to the wall are called shear walls. Houses with many rooms separated by structural walls with minimal openings are good examples of shear wall buildings. Figure below illustrates shear walls.
Braced Frame System
Braced frames act like shear walls but they are somewhat more flexible. In Figure below the dotted lines show the normal position of a shear wall and a braced frame. The braced frame is more flexible and bends farther from its normal position than the shear wall.
Moment-Resistant System
This system helps a building resist horizontal (lateral) forces at the joints between the columns and the beams. These joints become highly stressed, so they must be constructed of a strong, ductile material like steel. Figure below illustrates the moment-resistant system.
Dual Systems
Sometimes it is advantageous to use a combination of a moment-resistant frame and a shear wall or braced frame. This combination is called a dual system. The resisting systems (floors and walls) we have just covered are basic architectural components used in many buildings. In designing a seismically resistant building, the designer must choose some combination of these elements that will provide proper horizontal support. The choices the designer makes will have a major impact on the seismic safety, cost, and architecture of a building. SOME IMPORTANT TIPS FOR EARTHQUAKE RESISTANT DESIGH
Building should be of regular shapes. Cylindrical structures perform better in high-wind areas. Architect should try to design the building as aerodynamic as possible. This reduces the effect of Wind load on tall structures. There should no odd shapes in elevation and the whole building should be in balance. The center of gravity of building should not move. Cantilever projections should be minimum and their length should not be more than 3 to 4 feet. The span between the columns should be as small as possible. Point loads on load-carrying beams should be avoided.
The dead loads on the cottage-building should not be increased unnecessarily. For Example, Terrace garden or terrace swimming pools should be avoided, if possible. The sunk portions of WC and bath should be minimum.
Building should be a Reinforced Concrete framed structure. It provides better stability and reliability in Earthquake-prone areas. Cottage-building’s foundation should be placed on hard and level ground. There should not be very large overhead water tanks than are required. If it has to have larger capacity, then it should be divided into two three smaller tanks and should be kept at different locations to maintain balance of cottage-building. If the column length is more than 12 feet, then bracing beams should be provided in between the column at regular intervals. Bracing beams strengthen a column, and allow construction of multistoried buildings. The columns should be connected at each level.
For strengthening the brickwork, a sill or a lintel should be provided at every 3 feet level, and R.C.C. wall should be taken where it is possible. Cottage- building should not contain very large and heavy windows. They are bound to weaken the structure. The cottage- building’s electrification should contain a main switch and circuit breakers so as to avoid fire hazards because of short circuit in the earthquake. The glass used any structure should be fiber-reinforced glass or wire glass. Use of new and better materials like Fiber-reinforced Concrete and fiber-glass should be recommended. These new materials decrease dead load and increase the structure’s strength. If we manage to construct our buildings this way, we will be capable to fight the Earthquake and preventing the trail of loss of life and property that an Earthquake leaves behind.
SOMEOTHER TIPS TO REDUCE EFFECT OF EARTHQUAKE
Base Isolation
Seismic Dampers
CONCLUSION
To be earthquake proof, buildings, structures and their foundations need to be built to be resistant to sideways loads. The lighter the building is, the less the loads. This is particularly so when the weight is higher up. Where possible the roof should be of light-weight material. If there are floors and walls and partitions, the lighter these are the better, too. If the sideways resistance is to be obtained from walls, these walls must go equally in both directions. They must be strong enough to take the loads. They must be tied in to any framing, and reinforced to take load in their weakest direction. They must not fall apart and must remain in place after the worst shock waves so as to retain strength for the after shocks.
If the sideways resistance comes from diagonal bracing then it must also go equally all round in both directions. Where possible, it should be strong enough to accept load in tension as well as compression: the bolted or welded connections should resist more tension than the ultimate tension value of the brace (or well more than the design load) and it should not buckle with loads well above the design load. And the loads have got to go down to ground in a robust way. If the sideways load is to be resisted with moment resisting framing then great care has to be taken to ensure that the joints are stronger than the beams, and that the beams will fail before the columns, and that the columns cannot fail by spalling if in concrete. Again the rigid framing should go all around, and in both directions.
If the building resistance is to come from moment resisting frames, then special care should be taken with the foundation-to-first floor level. If the requirement is to have a taller clear height, and to have open holes in the walls, then the columns at this level may have to be much stronger than at higher levels; and the beams at the first floor, and the columns from ground to second floor, have to be able to resist the turning loads these columns deliver to the frame.
Alternatively, and preferably, the columns can be given continuity at the feet. This can be done with âfixed feetâ with many bolts into large foundations, or by having a grillage of steel beams at the foundation level able to resist the column moments. Such steel grillage can also keep the foundations in place. If the beams in the frame can bend and yield a little at their highest stressed points, without losing resistance, while the joints and the columns remain full strength, then a curious thing happens: the resonant frequency of the whole frame changes. If the building was vibrating in time with shock waves, this vibration will tend to be damped out. This phenomenon is known as âplastic hingeingâ and is easily demonstrated in steel beams, though a similar thing can happen with reinforced concrete beams as long as spalling is avoided.
All floors have to be connected to the framing in a robust and resilient way. They should never be able to shake loose and fall. Again all floors should be as light as possible. They should go all round each column and fix to every supporting beam or wall, in a way that cannot be shaken off. One way of reducing the vulnerability of big buildings is to isolate them from the floor using bearings or dampers, but this is a difficult and expensive process not suitable for low and medium rise buildings and low cost buildings. (Though it may be a good technique for Downtown Tokyo). Generally it is wise to build buildings that are not too high compared to their width in Earthquake areas, unless special precautions are taken.