Why Do Offspring Differ from Their Parents?
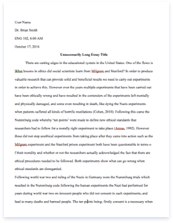
A limited time offer! Get a custom sample essay written according to your requirements urgent 3h delivery guaranteed
Order NowOffspring differ somewhat from their parents and from one another. Instructions for development are passed from parents to offspring in thousands of discrete genes, each of which is now known to be a segment of a molecule of DNA. This essay will explore some of the reasons behind how and why these differences in appearance arise, from the base sequence of DNA through to the observed phenotype. Genes come in different varieties, called alleles. Somatic cells contain two alleles for every gene, with one allele provided by each parent of an organism. Genotype refers to the information contained in an organisms DNA, or genetic material. Its phenotype is the physical expression of its genotype. Although every creature is born with a fixed genotype, the phenotype is a variable influenced by many factors in the animal’s environment and development. For example, two cows with identical genotypes could develop quite different phenotypes if raised in different environments and fed different foods. The close association of environment with the expression of the genetic information makes animal breeding a challenging endeavor, because the physical traits a breeder desires to selectively breed for cannot always be attributed entirely to the animal’s genes.
Moreover, most traits are due not just to one or two genes, but to the complex interplay of many different genes. DNA consists of a set of chromosomes; the number of chromosomes varies between species (humans, for example, have 46 chromosomes). Mammals (and indeed most creatures) have two copies of each chromosome in the DNA (this is called diploid). This means there are two copies of the same gene in an animal’s DNA. Sometimes each of these will be partially expressed. For example, in a person having one copy of a gene that codes for normal hemoglobin and one coding for sickle-cell hemoglobin, about half of the hemoglobin will be normal and the other half will be sickle-cell. In other cases, only one of the genes can be expressed in the animal’s phenotype. The gene expressed is called dominant, and the gene that is not expressed is called recessive. For instance, a human being could have two copies of the gene coding for eye color; one of them could code for blue, one for brown.
The gene coding for brown eyes would be dominant, and the individual’s eyes would be brown. But the blue-eyes gene would still exist, and could be passed on to the person’s children. Most of the traits an animal breeder might wish to select will be recessive, for the obvious reason that if the gene were always expressed in the animals, there would be no need to breed for it. If a gene is completely recessive, the animal will need to have two copies of the same gene for it to be expressed (in other words, the animal is homozygous for that particular gene). For this reason, animal breeding is usually most successful when animals are selectively inbred. If a bull has two copies of a gene for a desirable recessive trait, it will pass one copy of this gene to each of its offspring. The other copy of the gene will come from the cow, and assuming it will be normal, none of the offspring will show the desirable trait in their phenotype. However, each of the offspring will have a copy of the recessive gene. If they are then bred with each other, some of their offspring will have two copies of the recessive gene. If two animals with two copies of the recessive gene are bred with each other, all of their offspring will have the desired trait.
In order to make eggs and sperm, which are called gametes, a special kind of cell division occurs called meiosis, in which cells divide so that each one has half the normal number of chromosomes (in humans, each sperm and egg contains 23 chromosomes). Before this division occurs, the two pairs of chromosomes wrap around each other, and a phenomenon known as crossing over takes place in which sections of one chromosome will be exchanged with sections of the other chromosome so that new combinations are generated. The problem with crossing over is that some unexpected results can occur. For instance, the offspring of a bull homozygous for two recessive but desirable traits and a cow with “normal” genes will all have one copy of each recessive gene.
But when these offspring produce gametes, one recessive gene may migrate to a different chromosome, so that the two traits no longer appear in one gamete. Since most genes work in complicity with others to produce a certain trait, this can make the process of animal breeding very slow, and it requires many generations before the desired traits are obtained In meiosis the pairs of chromosomes (that code for possible outcomes of characteristics) temporarily join and exchange information (crossing over) creating different combinations of gene types (alleles). For example; a pair of chromosomes could be a dominant allele (gene type) and a recessive allele which might code for brown hair. After crossing over it might be recessive which could be blond hair.
Chromosomes line up in different orders (random assortment) so when they go to either new cell on cell might have dominant for one characteristic (e.g. brown eyes), recessive for another (e.g. blond hair), and dominant for a third one (e.g. can roll tongue) whereas the other created cell might be dominant, dominant dominant (brown eyes, brown hair and can roll tongue.
More rare is mutation where DNA is randomly altered (although certain things can make this more likely e.g. UV light).This is called genetic variation. Genetic variation occurs in species so in the event of a change in the environment, e.g. climate change, a new predator, new diseases etc, hopefully some of the species will survive. If we were all identical if one is killed by a disease we all would be. In sexual reproduction of plants and animals, a specialized cell from a female fuses with a specialized cell from a male. Each of these sex cells contains an unpredictable half of the parent’s genetic information. When a particular male cell fuses with a particular female cell during fertilization, they form a cell with one complete set of paired genetic information, a combination of one half-set from each parent. As the fertilized cell multiplies to form an embryo, and eventually a seed or mature individual, the combined sets are replicated in each new cell. This sorting and combination of genes in sexual reproduction results in a great variety of gene combinations in the offspring of two parents.
There are millions of different possible combinations of genes in the half apportioned into each separate sex cell, and there are also millions of possible combinations of each of those particular female and male sex cells. However, new mixes of genes are not the only source of variation in the characteristics of organisms. Although genetic instructions may be passed down virtually unchanged for many thousands of generations, occasionally some of the information in a cell’s DNA is altered. Deletions, insertions, or substitutions of DNA segments may occur spontaneously through random errors in copying, or may be induced by chemicals or radiation. If a mutated gene is in an organism’s sex cell, copies of it may be passed down to offspring, becoming part of all their cells and perhaps giving the offspring new or modified characteristics. Some of these changed characteristics may turn out to increase the ability of the organisms that have it to thrive and reproduce, some may reduce that ability, and some may have no appreciable effect. Evolution is the process of biological change over time. Such changes, especially at the genetic level are accomplished by a complex set of evolutionary mechanisms that act to increase or decrease genetic variation.
Evolution requires genetic variation, and these variations or changes (mutations) can be beneficial, neutral or deleterious. In general, there are two major types of evolutionary mechanisms, those that act to increase genetic variation, and mechanisms that operate to decrease genetic mechanisms. Mechanisms that increase genetic variation include mutation, recombination and gene flow. Mutations generally occur via chromosomal mutations, point mutations, frame shifts, and breakdowns in DNA repair mechanisms. Chromosomal mutations include translocations, inversions, deletions, and chromosome non-disjunction. Point mutations may be nonsense mutations leading to the early termination of protein synthesis, mutations that results and a substitution of one amino acid for another in a protein), or silent mutations that cause no detectable change. Recombination involves the re-assortment of genes through new chromosome combinations. Recombination occurs via an exchange of DNA between homologous chromosomes (crossing over) during meiosis. Recombination also includes linkage disequilibrium.
With linkage disequilibrium, variations of the same gene (alleles) occur in combinations in the gametes (sexual reproductive cells) than should occur according to the rules of probability. Gene flow occurs when individuals change their local genetic group by moving from one place to another. These migrations allow the introduction of new variations of the same gene (alleles) when they mate and produce offspring with members of their new group. In effect, gene flow acts to increase the gene pool in the new group. Because genes are usually carried by many members of a large population that has undergone random mating for several generations, random migrations of individuals away from the population or group usually do not significantly decrease the gene pool of the group left behind. In contrast to mechanisms that operate to increase genetic variation, there are fewer mechanisms that operate to decrease genetic variation. Mechanisms that decrease genetic variation include genetic drift and natural selection.
Genetic drift results form the changes in the numbers of different forms of a gene (allelic frequency) that result from sexual reproduction. Genetic drift can occur as a result of random mating (random genetic drift) or be profoundly affected by geographical barriers, catastrophic events (e.g., natural disasters or wars that significantly affect the reproductive availability of selected members of a population), and other political-social factors. Natural selection is based upon the differences in  the viability and reproductive success of different genotypes with a population (differential reproductive success). Natural selection can only act on those differences in genotype that appear as phenotypic differences that affect the ability to attract a mate and produce viable offspring that are, in turn, able to live, mate and continue the species. Evolutionary fitness is the success of an entity in reproducing (i.e., contributing alleles to the next generation). There are three basic types of natural selection. With directional selection an extreme phenotype is favored (e.g., for height or length of neck in giraffe).
Stabilizing selection occurs when intermediate phenotype is fittest (e.g., neither too high or low a body weight) and for this reason it is often referred to a normalizing selection. Disruptive selection occurs when two extreme phenotypes are fitter that an intermediate phenotype. Natural selection does not act with foresight. Rapidly changing environmental conditions can, and often do, impose new challenges for a species that result in extinction. In addition, evolutionary mechanisms, including natural selection, do not always act to favor the fittest in any population, but instead may act to favor the more numerous but tolerably fit. Thus, the modern understanding of evolutionary mechanisms does not support the concepts of social Darwinism. The operation of natural evolutionary mechanisms is complicated by geographic, ethnic, religious, and social groups and customs. Accordingly, the effects of various evolution mechanisms on human populations are not as easy to predict. Increasingly sophisticated statistical studies are carried out by population geneticists to characterize changes in the human genome.