Antibiotic Resistance
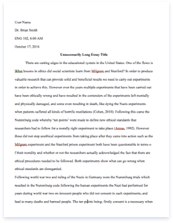
- Pages: 20
- Word count: 4984
- Category: Gene
A limited time offer! Get a custom sample essay written according to your requirements urgent 3h delivery guaranteed
Order NowAbstract
The spread of antibiotic resistance is a universal threat to both humans and animals for treatment of microbial infections. The antibiotic resistance is generally not preventable but can still be controlled. Prolonged and repeated use of antibiotic leads to many pathogen becoming resistant to antibiotics. The resistance may be either intrinsic or acquired depending on the condition. Although classically attributed to chromosomal mutations, resistance is most commonly associated with extra-chromosomal elements acquired from other bacteria in the environment.
These include different types of mobile DNA segments, such as plasmids, transposons, and integrons. However, intrinsic mechanisms not commonly specified by mobile elements such as efflux pumps that expel multiple kinds of antibiotic are now recognized as major contributors to multidrug resistance in bacteria. Once established, multidrug resistance is a worldwide problem that does not obey international borders and can indiscriminately affect members of all socioeconomic classes. So, the issue of antibiotic resistant is one the most urgent priorities to overcome in the field of Human Health Science.
Keywords: Antibiotic, intrinsic resistance, acquired resistance, mutation, plasmid, intergorns, efflux pump.
INTRODUCTION
It is said that the invention of the antibiotic is the most pioneer achievement of humans against the bacterial infections in the human health science. The first antibiotic appeared in 1928 by Alexander Flaming that is penicillin which kill all surrounding bacteria. And thus, the concept of antibiotic came and it changed the method of infection treatment. The word âAntibioticâ was first termed by the Selman Waksman. But the problem came that is Antibiotic resistance. As a natural response, antibiotic resistance emerges in pathogen populations. Resistance is a condition in which the antibiotic fails to harm the pathogen enough to cure disease. Emergence of resistance often begins with a large pathogen population in which a tiny fraction is naturally resistant to the antibiotic, either through spontaneous changes or through the acquisition of resistance genes from other microbes. Antibiotic treatment kills or halts the growth of the major, susceptible portion of the microbial population (Abraham E.P, 1940).
That favors growth of resistant mutants. Prolonged, repeated use of a particular antibiotic leads to the bulk of the pathogen population being composed of resistant cells. Subsequent treatment with that antibiotic does little good. If the resistant organisms spread to other persons, the resulting infections are resistant before treatment. Control of such infection requires a different antibiotic. The development of resistance is accelerated by the mutagenic action of some antibiotics, by the movement of resistance genes from one microbial species to another, and by our excessive, inappropriate use of antibiotics. A wide range of biochemical and physiological mechanisms may be responsible for resistance (Levy et al., 2004). The lack of basic knowledge on these topics is one of the primary reasons that there has been so little significant achievement in the effective prevention and control of resistance development. Most international, national, and local agencies recognize this serious problem.
Many resolutions and recommendations have been propounded, and numerous reports have been written, but to no avail: the development of antibiotic resistance is relentless (Davies J. et al.2010). For example, S. aureus was susceptible for many antibiotics including penicillin, before seventy years. But this susceptibility disappeared after few years. Methicillin, which overcame resistance to penicillin. But in 1960, one year after the introduction of methicillin, MRSA (Methicillin Resistant Staphylococcus aureus ) was recovered in the United Stat. So, the bacteria resist quickly but their loss of resistance rate is very slow. Multiply resistant organisms render therapy more precarious and costly and sometimes unsuccessful. Individuals may succumb to MDR infections because all available drugs have failed, especially in the developing world (DeLeo et al., 2009). Notable global examples include hospital and community MDR strains of Mycobacterium tuberculosis, Enterococcus faecium, Enterobacter cloacae, Klebsiella pneumoniae, S. aureus, Acinetobacter baumanii and Pseudomonas aeruginosa.
History of Antibiotic Resistance
Resistance to single antibiotics became prominent in organisms that encountered the first commercially produced antibiotics. The most notable example is resistance to penicillin among staphylococci, specified by an enzyme (penicillinase) that degraded the antibiotic (Abraham E.P, 1940). In the case of streptomycin, introduced in 1944 for the treatment of tuberculosis mutant strains of Mycobacterium tuberculosis resistant to therapeutic concentrations of the antibiotic were found to arise during patient treatment ( Kang et al., 2005). 1959, the Japanese found Shigella species that were resistant to Sulfonamides, Streptomycin, Chloramphenicol, and Tetracycline. The resistance was due to plasmid, which carried different antibiotic resistance genes. The other MDR mechanism is due to sequential accumulation of chromosomal mutations in different drug resistant genes, as in the case of MDR-TB and XDR-TB (Miller et al.,2005). The most prevalent Gram-negative pathogens, such as Escherichia coli, Salmonella enterica, and Klebsiella pneumonia cause a variety of diseases in humans and animals, and a strong correlation between antibiotic use in the treatment of these diseases and antibiotic resistance development has been observed over the past half-century.
This is especially apparent with the -lactam class of antibiotics and their related inactivating enzymes, the -lactamases. At this time, several groups and classes have been identified, comprising up to 1,000 resistance related -lactamases. In 2008, NDM-1 (New Delhi Metallobeta-lactamase-1) is an enzyme that makes bacteria to resist to a broad range of beta-lactam antibiotic. The infection caused by it is difficult to treat. It was first detected in K. pneumonia isolate from a Swedish patient of Indian origin in 2008. (Kumarasamy et. al., 2010). Due the this NDM-1, In the RIO DE JANERIO 108 cases were identified and 18 patients have died,the reports said. Currently, the most notorious superbug is the Gram-positive organism Staphylococcus aureus.
Whether it is the most serious superbug can be debated, since one wonders to what extent its bad reputation is due to its extensive press coverage. S. aureus has a close association with humankind: it is carried as a nasal commensal in 30% of the population, and its presence has long been linked to common skin infections such as boils (Enright et.al.,2002). Currently, it is estimated that more than 70% of the bacteria that cause hospital-acquired infections are resistant to at least one of the antibiotic used to treat them.
Molecular and cellular mechanism of antibiotic resistance
Antibiotic resistance can be divided into natural resistance and acquired resistance. Natural resistance means that the bacteria are âintrinsicallyâ resistant. For example, Streptomyces has some genes responsible for resistance to its own antibiotic. Other examples include organisms that lack a transport system or a target for the antibiotics. In other cases, the resistance can be due to increased efflux activity. Acquired resistance refers to bacteria that are usually sensitive to antibiotics, but are liable to develop resistance. Acquired resistance is often caused by mutations in chromosomal genes, or by the acquisition of mobile genetic elements, such as plasmids or transposons, which carry the antibiotic resistance genes (Russell A.D. et al., 1996). Understanding the mechanisms of resistance has become a significant biochemical issue over the past several years and nowadays there is a large pool of information about how bacteria can develop drug resistance. Biochemical and genetic aspects of antibiotic resistance mechanisms in bacteria are shown in Figure: 1.
Figure: 1. Aspects of antibiotic resistance mechanism (DĆŸidic et. al., 2007)
BICHEMICAL ASPECTS
There is an amazing diversity of antibiotic resistance mechanisms within each of these categories and a single bacterial strain may possess several types of resistance mechanisms. Which of these mechanisms prevails depends on the nature of the antibiotic, its target site, the bacterial species and whether it is mediated by a resistance plasmid or by a chromosomal mutation.
1. ANTIBIOTIC INACTIVATION
In this mechanism, organism produces some enzymes that degrade or modify the drug itself and that organism will become resist to that antibiotic. Biological strategies such as hydrolysis, group transfer and redox mechanism.
A) Hydrolysis
Many antibiotics have hydrolytically susceptible chemical bonds (e.g. esters and amides). Some organisms produce such type of enzymes which can destroy antibiotic by targeting and cleaving these bonds. So, these enzymes are secreted by organism and it inactivate the antibiotic, before it reached its target site. Beta-lactamase is the best example of hydrolytic enzyme that cleaves the beta-lactam ring of the penicillin and cephalosporin antibiotics. Many Gram-negative and Gram-positive bacteria produce such enzymes, and more than 200 different ÎČ-lactamases have been identified. ÎČ-lactamases are classified into four groups on the basis of functional characteristics, including preferred antibiotic substrate. Clinical isolates often produce ÎČ-lactamases belonging to different functional groups. They are most commonly detected in Escherichia coli, Klebsiella pneumonia and Proteus mirabilis, but have also been found in other Enterobacteriaceae (Bradford P.A. 2001).
B) Group transfer
The most diverse family of resistant enzymes is the group of transferases. By the chemical process such as adenylyl, phosphoryl or acetyl groups are added to the periphery of the antibiotic molecule and thus resistant enzymes inactivate the antibiotics (Vetting M.W. 2004). So, structure of antibiotics will be affected and it will lose its target site for binding. These covalent modification strategies all require a co-substrate for their activity consequently these processes are restricted to the cytoplasm.
C) Redox process
The oxidation or reduction of antibiotics has been infrequently exploited by pathogenic bacteria. However, there are a few of examples of this strategy. One is the oxidation of tetracycline antibiotics by the TetX enzyme. Streptomyces virginiae, producer of the type A streptogramin antibiotic virginiamycin M1, protects itself from its own antibiotic by reducing a critical ketone group to an alcohol at position 16.
Figure 2: Mechanism of resistnce. (J. Wiley,2004.)
2. TARGET MODIFICATION
In this mechanism, the organism modify the target site and thus antibiotic unable to bind properly. The vital cellular function the target site, microbes are not affected by antibiotic action by dispensing with them entirely. However, it is possible for mutational changes to occur in the target that reduce susceptibility to inhibition. In some cases, the modification in target structure needed to produce resistance requires other changes in the cell to compensate for the altered characteristics of the target.
A) Peptidoglycan structure alteration
Peptidoglycan, the main component of the call wall of bacteria is the best site for antibiotic for inhibition. The antibiotic target the enzymes involving the peptidoglycan synthesis but bacteria will change the structure of that enzyme and it become resist. The presence of mutations in the penicillin-binding domain of penicillin-binding proteins (PBPs) results in decreased affinity to b-lactam antibiotics (.Tenover F.C , 2006). Alterations among PBPs result in ampicillin resistance among Enterococcus faecium, and penicillin resistance among Streptococcus pneumonia. Glycopeptides such as vancomycin inhibit cell wall of Gram-positive bacteria by binding C-terminal acyl-D-alanyl-D-alanine (acyl-D-Ala-D-Ala)-containing residues in peptidoglycan precursors. Resistance is achieved by altering the target site by changing the D-Ala-D-Ala to D-alanyl-D-lactate (D-Ala-D-Lac) or D-alanyl-D-serine (D-Ala-D-Ser) at the C-terminus, which inhibits the binding of vancomycin.
B) Protein synthesis interference
Many antibiotics target the protein synthesis process to kill the bacteria. But for resistnce from antibiotic, they interfere in protein synthesis or transcription via RNA polymerase is achieved by the modification of specific target. Many groups of antibiotic like macrolide , streptogramin B block the protein synthesis by the binding with 50s ribosome subunit. Resistance to this antibiotics, mainly Gram positive bacteria modify the 23s subunit of 50s ribosome. Mutations in 23S rRNA close to the sites of methylation have also been associated with resistance to the macrolide group of antibiotics in a range of organisms. In addition to multiple mutations in the 23S rRNA, alterations in the L4 and L22 proteins of the 50S subunit have been reported in macrolide-resistant S. pneumonia(Dzidic S. et al.,2008).
C) DNA synthesis interference
Some antibiotics target the enzymes involving in DNA replication. Fluoroquinolones interact with the DNA gyrase and topoisomerase IV enzymes and prevent DNA replication and transcription. Resistance carried by the mutation the in some region of target enzyme and it altered the site. Antibiotic failed to inhibite that microorganism.
3. EFFULX PUMPS AND OUTER MEMBRANE PERMIABILITY
The efflux pumps are the membrane proteins that export the antibiotics out of the cell and keep its intracellular concentrations at low levels. Reduced outer membrane (OM) permeability results in reduced antibiotic uptake. The reduced uptake and active efflux induce low level resistance in many clinically important bacteria.
A) Efflux pump
Efflux pumps are the membrane bound proteins which throw out the undesired molecules from the cell. Efflux pumps affect all classes of antibiotics, especiallythe macrolides, tetracyclines, and fluoroquinolones because these antibiotics inhibit different aspects of protein and DNA biosynthesis and therefore must be intracellular to exert their effect. Although some are drug-specific, many efflux systems are multidrug transporters that are capable of expelling a wide spectrum of structurally unrelated drugs, thus contributg significantly to bacterial multidrug resistance (MDR). Inducible multidrug efflux pumps are responsible for the intrinsic antibiotic resistance of many organisms, and mutation of the regulatory elements that control the production of efflux pumps can lead to an increase in antibiotic resistance (Nikaido H.,1994).
For example, the MexAB-OprM efflux pump in Pseudomonas aeruginosa is normally positively regulated by the presence of drugs, but mutations in its regulator (mexR) lead to the overexpression of MexAB-OprM, which confers increased resistance to antibiotics such as beta-lactams. Recently, a large tandem of the E. coli acrAB locus in a mutant isolated in the presence of tetracycline was found to overexpress the AcrAB drug efflux pump, producing an MDR phenotype Efflux transporters can be further classified into single or multicomponent pumps. Single component pumps transport their substrates across the cytoplasmic membrane(Webber et al.,2003). Multicomponent pumps, found in Gram-negative organisms, function in association with a periplasmic membrane fusion protein (MFP) component and an outer membrane protein (OMP) component, and efflux substrates across the entire cell envelope.
B) Outer membrane (OM) permeability changes
Gram negative bacteria possess a outer membrane consisting of an outer layer contains lipopolysaccharide and inner layer contain phospholipid. So, the entry of antibiotic is slow down due to this outer membrane and transport across the OM is achieved by porin proteins that form water-filled channels. Drug molecules can penetrate the OM employing one of the following modes: by diffusion through porins, by diffusion through the bilayer. The mode of entry employed by a drug molecule largely depends on its chemical composition (Tenover, F.C. 2006). For example, hydrophilic compounds either enter the periplasm through porins. Antibiotics such as beta-lactams, chloramphenicol and fluoroquinolones enter the Gram-negative outer membrane via porins. As such, changes in porin copy number, size or selectivity will alter the rate of diffusion of these antibiotics. The mutation in the lipopolysaccharides, resist the entry of antibiotics. Strains of E. coli and S. enterica defective in lipopolysaccharides
Figure 3: mechanism of resistance (J. Wiley, 2004)
have been found to be at least 4-fold more susceptible toerythromycin, roxithromycin, clarithromycin and azithromycinthan the wild-type strains( Nikaido H.,1994).
GENETICAL ASPECTS OF ANTIBIOTIC RESISTANCE
Genetic determinants for resistance are found in various pathogenic bacteria. Resistance can be either intrinsic property or acquired. Acquired bacterial antibiotic resistance can result from a mutation of cellular genes, the acquisition of foreign resistance genes or a combination of these two mechanisms. Thus, there are two main ways of acquiring antibiotic resistance: 1.Through mutation in different chromosomal loci and 2.
Horizontal gene transfer.
1. MUTATION
Any changes in the genetic material and which can pass from generation to generation known as mutation.
A) Spontaneous mutations
In the replication error or in DNA repair mechanism this mutation can occur in actively diving cells. They are called growth dependent mutations and present an important mode of generating antibiotic resistance. By nucleotide point mutation, the resistance occurs and it able to produces resistant phenotype. Each pathway requires the gene expression and these variety of gene involve in antibiotic resistance for protection of bacterial cell. There are a substantial number of biochemical mechanisms of antibiotic resistance that are based on mutational events, like the mutations of the sequences of genes encoding the target of certain antibiotics. The uptake system of antibiotic and efflux system can be modified by the mutation in regulatory system or in promoter region.
The over expression of the efflux system may confer the multiple drug resistance. Many Gram-negative microorganisms produce chromosomal beta-lactamases at low levels and mutations producing up-regulation of their expression may lead to resistance to most cephalosporins. P. aeruginosa from the lungs of patients with cystic fibrosis is almost impossible to eradicate, mainly because of the development of resistance to multiple antibiotics (Martinez, 2000). In this particular environment resistance is achieved through chromosomal mutations that are able to produce resistance to all antibiotics used in clinical practice, without any acquisition of exogenous DNA.
B) Hypermutators
Low spontaneous mutation rates are maintained by the activity of many molecular mechanisms that protect and repair DNA, as well as by the mechanisms that assure high-fidelity of DNA replication .However, bacteria with hypermutable strains among natural and laboratory populations have been found. Experimental studies indicate that the frequency of mutators observed among natural and clinical bacterial isolates is much higher than expected, which suggests that there are situations in nature where being a mutator confers a selective advantage. According to the currently most acceptable hypermutable stateâ model, during a prolonged non-lethal antibiotic selective pressure a small bacterial population enters a transient state of a high mutation rate.
Hypermutators have been found in populations of E. coli, Salmonella enterica, Neisseria meningitidis, Haemophilus influenzae, Staphylococcus aureus, Helicobacter pylori, Streptococcus pneumoniae, P. aeruginosa with frequencies ranging from 0.1 to above 60 %. By increasing the possibility of mutations, they may accelerate the evolution of favorable mutations under certain conditions. During this process, mutators can be fixed in the population by getting along with the favourable mutations, they have created. Thus, the acquisition of a mutator phenotype may increase the chance of acquiring antibiotic resistance by mutational events. Hypermutators may also enable multiresistant phenotype (Martinez, 2000).
C) Adaptive mutagenesis
Mutations arise also in non-dividing or slowly dividing cells and have some relation to the selective pressure used and occurs under natural condition. These mutations, named âadaptive mutationsâ, arise only in the presence of non-lethal selective pressure that favours them. This is the main feature that distinguishes them from the growth dependent, spontaneous mutations.
2. HORIZONTAL GENE TRANSFER
The transfer of genetic material horizontally may lead the antibiotic resistance in the bacteria. Resistance genes may transfer by the mechanism of conjugation, transformation and transduction. Resistance genes can be further incorporated into the recipient chromosome by recombination. These genes may contain single mutations or more severe sequence changes. Conjugation is mediated by a particular kind of circular DNA called plasmid, replicates independently of the chromosome. This plasmid may transfer into other bacterial cell by the process of conjugation. Plasmid may carry the resistance gene. Gram-negative anaerobes and Gram-positive bacteria, conjugative transposons are recognized as important mediators of genetic exchange on a par with the large R-plasmids of enteric bacteria. These large (>25 kb) elements encode a fully functional conjugation apparatus and are capable of self-transfer to a wide variety of species.
Conjugative transposons in the Bacterioides are referred to as tetracycline resistance elements owing to the presence of tetracycline resistance genes (tetQ) and these elements are primarily responsible for more than 80 % of tetracycline resistance frequency among Bacteroides clinical isolates. The gene conferring this phenotype has been associated with both narrow and broad host range plasmids. The nature of these conjugative elements raises the possibility of the resistance gene spreading to other pathogenic bacteria. Transduction is mechanism in which virus serves as the agent of transfer between bacterial strains. Transformation is mechanism in which DNA released from a bacterium is picked up by a new cell. After the new DNA is introduced whether via conjugation, transduction, or transformation it is incorporated into the cell and results in the emergence of a new, resistant genotype.
Figure 4: Gene Transfer mechanism.( J.Wiley,2004).
A) Integrons
Horizontal transfer of resistance genes a mechanism the dissemination of multiple drug resistance because resistance genes can be found in clusters and transferred together to the recipient. This is enabled by the existence of specific DNA structures called integrons. Integrons are DNA elements with the ability to capture genes, notably those encoding antibiotic resistance, by site-specific recombination. These elements are located either on the bacterial chromosome or on broad host range plasmids. Integrons differ from transposons in two important characteristics: transposons have direct or indirect repeat sequences at their ends, but the regions surrounding the antibiotic resistance genes in the integrons are not repeats; and the integrons contain a site specific integrase gene of the same family as those found in phages but lack gene products associated with transposition (Mazel, D. 2006). Integrons promote the capture of one or more gene cassettes within the same attachment site thereby forming clusters of antibiotic resistance genes. Gene cassettes are the smallest mobile genetic entities that can carry resistance determinants.
These can encode many types of resistance including to trimethoprim, chloramphenicol, b-lactams, aminoglycosides,and quinolones and for each of these antibiotic classes several distinct gene cassettes have been reported. Resistance gene cassettes have been found for the most classes of antibiotics, and the gene products are involved in various mechanisms of resistance, such as efflux, target bypass and drug inactivation. Over 40 gene cassettes and three distinct classes of integrons have been identified (Dzidic et al.,2008). Integron movement allows transfer of the cassett associated resistance genes from one DNA replicon to another. Horizontal transfer of the resistance genes can be achieved when an integron is incorporated into a broad host range plasmid.
A plasmid with a pre-existing resistance gene cassette can acquire additional resistance gene cassettes from donor plasmids, thus spreading multiresistance. All resistance-encoding DNAs establish a resistance gene pool, which represents a potential source for the horizontal transfer between bacteria. (Tenover, 2006). Studies about horizontal gene transfer-emerging multidrug resistance in hospital bacteria have demonstrated that the transfer of antibiotic resistance genes can take place in the intestine between Gram-positive or Gram-negative bacilli. The fact that bacteria that have been separately evolving for up to 150 million years can exchange DNAs has strong implications with regard to the evolution of antibiotic resistance in bacterial pathogens.
Ecology of Antibiotic resistance
The impact of the drug selection process can be largely confined to the individual taking the antibiotic if widespread antibiotic usage is absent. After therapy, the selected resistant commensal strains will eventually be âdiluted outâ and their growth will be suppressed by the return of drug-susceptible, natural competitors. If, however, whole populations are being treated with the same class of antibiotic, susceptible strains will have little opportunity to recolonize their niche and resistant strains will acquire an important advantage. The resulting ecological imbalance produces a potentially serious environmental pool of resistance genes. Ecologically speaking, it is the density of antibiotic usage that enhances resistance selection and its effects.
The âselection densityâ involves the total amount of antibiotic being applied to a geographically defined number of individuals in a setting, whether it is the home, daycare center, hospital or farm. Each individual becomes a âfactoryâ of resistant bacteria that enter the environment (Levy, 1997). The disparity between resistance rates in the local community and those in city hospitals reflects differential ecological effects of antibiotic use. In recent times, studies of microbes in natural environments have provided drastically altered concepts of the natural lifestyles and functions of bacteria and questions have been asked about the possible roles of the large number of bioactive microbial compounds that are produced.
HOW TO MANAGE THE ANTIBIOTIC RESISTANCE PROBLEM
1) Limiting the spread of drug resistant bacteria
Several measures could be used to prevent the spread of drug resistant bacteria. First, we could use better treatment strategies; better immunization programmes; improved hygiene and nutrition; and initiatives targeting the poor populations. Second, it might be useful to establish antibiotic resistance surveillance programmes. Third, better education of health care professionals is required to prevent the prescription of unnecessary antibiotics. It is noteworthy that significant investment of time, effort, and money is necessary in order to control antibiotic resistant bacteria. Of course, as long as antibiotics are used, antibiotics resistance is bound to occur. However, we might be able to reduce the drug resistance problem. One strategy is to ensure that antibiotics are used only when necessary. A second strategy is to ensure that they are used for time; that is, that the treatment is not stopped before it is completed. Patient compliance is a key problem in that respect. A third strategy for limiting the appropriate amount of drug resistance is to use antibiotics combinations. Unfortunately, while all these strategies seem sound in theory, in reality, the problem persists.
2) Development of new antibiotics
Another possibility is to develop new antibiotics. However, that is not an easy task. The sad irony is that many pharmaceutical companies have decided to abandon their antibiotic development programmes when new antibiotics are needed most, since 99% of the drug candidates fail, and antibiotics are not as profitable as other, more commonly used, drugs. The traditional approach of screening microbes for antibiotics is not efficient. So, we need the new era for research for antibiotic. A second approach, which utilises target-based screening, became popular when genomics tools became available. However, although the idea is appealing, in reality, it is extremely difficult. Many companies have tried this approach, and so far they have all failed. The whole organism-based approach is more feasible but the conditions of screen need careful consideration.
3) Phage therapy
Phage therapy can also be used to deal with antibiotics resistance. This approach had already been used by the Russians during the Second World War, and has been gaining popularity again in recent years. Phage can be applied on the wounds of a patient to kill the bacteria, and has proven to be quite effective. Of course, it cannot be used for internal infections, and the bacteria might also develop phage resistance.
4) Mobilization of host defense mechanisms
Yet another approach is to mobilize host defense mechanisms. This can be achieved through the mobilization of innate immunity such as defensins, or through the development of vaccines, which make antibiotics less necessary. The idea is to boast the immune response capability to control the bacterial infection. Of course, that approach is not always successful (Levy,1997).
5) The use of normal bacterial flora
Finally, one could also potentially use normal bacterial flora to suppress some pathogens.
CONCLUSION
It is clear that bacteria will continue to develop resistance to currently available antibacterial drugs by either new mutations or the exchange of genetic information, that is, putting old resistance genes into new hosts. In many healthcare facilities around the world, bacterial pathogens that express multiple resistance mechanisms are becoming the norm, complicating treatment and increasing both human morbidity and financial costs. Prudent use of antibacterial drug using the appropriate drug at the appropriate dosage and for the appropriate duration is one important means of reducing the selective pressure that helps resistant organisms emerge.
The other vital aspect of controlling the spread of multidrug-resistant organisms is providing sufficient personnel and resources for infection control in all healthcare facilities. New antibacterial agents with different mechanisms of action are also needed. It is difficult to outsmart organisms that have had several billion years to learn how to adapt to hostile environments, such as those containing antimicrobial agents. Yet, with sufficient efforts to use antimicrobial agents wisely, thereby preventing the emergence of resistant organisms, and strict attention to infection control guidelines to contain the spread of resistant organisms when they develop, we should be able to stay at least one step ahead of the next resistant plague.
REFERENCES:
1. Levy, S. B., and B. Marshall. 2004. Antibacterial resistance worldwide: causes, challenges and responses. Nat. Med. 10(Suppl.):S122âS129. 2. Abraham EP, Chain E (1940).An enzyme from bacteria able to destroy penicillin. Nature 46(3713):830-837. 3. Julian Davies and Dorothy Davies. Origins and Evolution of Antibiotic Resistance. Microbiology and molecular biology reviews, Sept. 2010, p. 417â433 4. DeLeo, F. R., and H. F. Chambers. 2009. Reemergence of antibiotic-resistant Staphylococcus aureus in the genomics era. J. Clin. Invest. 119:2464-2474. 5. Kang CI, Kim SH, Park WB, et al. Bloodstream infections caused by antibiotic-resistant gram-negative bacilli: risk factors for mortality and impact of inappropriate initial antimicrobial therapy on outcome. Antimicrob Agents Chemother. 2005;49:760 â766. 6. Kumarasamy KK, Toleman MA, Walsh TR et al., august 2010. Emergence of a new antibiotic resistance mechanism : a molecular, biological, and epidemiological study. Lancet Infect dis (9):597-602. 7. Enright, M. C., D. A. Robinson, G. Randle, E. J. Feil, H. Grundmann, and B. G. Spratt. 2002. The evolutionary history of methicillin-resistant Staphylococcus aureus (MRSA). Proc. Natl. Acad. Sci. U. S. A. 99:7687â7692. 8. A.D. Russell, I. Chopra (Eds.), Understanding Antibacterial Action and Resistance, Ellis Horwood, Hertfordshire, UK (1996). 9. S. Dzidic et al.: Antibiotic Resistance in Bacteria, Food Technol. Biotechnol. 46 (1) 11â21 (2008) 10. P.A. Bradford, Extended-spectrum b-lactamases in the 21stcentury: Characterization, epidemiology, and detection ofthis important resistance threat, Clin. Microbiol. Rev. 14 (2001) 933â951. 11. M.W. Vetting, S. Magnet, E. Nieves, S.L. Roderick, J.S. Blanchard, A bacterial acetyltransferase capable of regioselective N-acetylation of antibiotics and histones, Chem. Biol. 11 (2004) 565â573.
12. F.C. Tenover, Mechanisms of antimicrobial resistance in bacteria, Am. J. Med. (Suppl.), 119 (2006) 3â10. 13. H. Nikaido, H.I. Zgurskaya, Antibiotic efflux mechanisms, Curr. Opin. Infect. Dis. 12 (1999) 529â536. 14. M.A. Webber, L.J. Piddock, The importance of efflux pumps in bacterial antibiotic resistance, J. Antimicrob. Chemother. 51 (2003) 9â11. 15. J.L. Martinez, F. Baquero, Mutation frequencies and antibiotic resistance, Antimicrob. Agents Chemother. 44 (2000) 1771â1777. 16. Mazel, D. 2006. Integrons: agents of bacterial evolution. Nat. Rev. Microbiol. 4:608â620. 17. Levy, S.B. Antibiotic resistance: an ecological imbalance, in Antibiotic Resistance: Origins, Evolution, and Spread, 1â9 (J. Wiley, Chichester, UK, 1997). 18. American Academy of Microbiology. 2005. Vaccine development: current status and future needs. Based on a colloquium held in Washington, DC, 4 to 6 March 2005. ASM Press, Washington, DC.